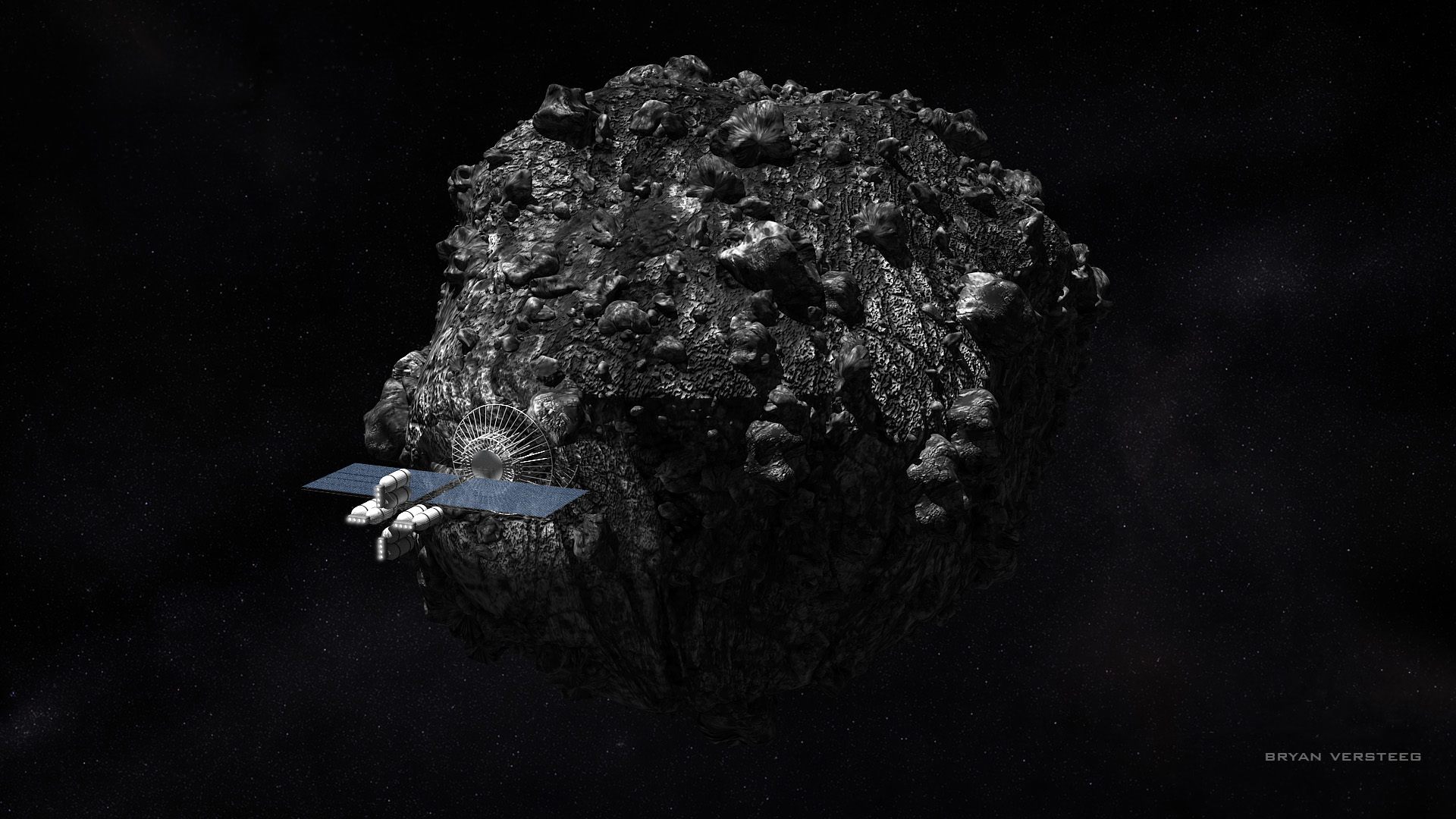
Publications + Presentation
NEAR EARTH OBJECTS AS RESOURCES FOR SPACE INDUSTRIALIZATION
MARK SONTER
Consultant, Asteroid Enterprises Pty Ltd,
28 Ian Bruce Crescent, Balgownie, New South Wales 2519, Australia
INTRODUCTION:
The Near Earth Asteroids are potential impact threats to Earth, but also a particularly accessible subset of them does provide potentially attractive targets for resources to support space industrialization. Robust technical and economic approaches to evaluation of the feasibility of proposed projects are necessary for assessment of such space mining ventures. This paper discusses the technical engineering and mission–planning choices and shows how the concept of probabilistic Net Present Value can be used to optimize these choices, and hence select between alternative asteroid mining mission designs
The generic mission reviewed envisages a lightweight remote (teleoperated) or semi autonomous miner, recovering products such as water or nickel-iron metal, from highly-accessible NEAs, and returning it to Low Earth Orbit (LEO), for sale and use in LEO, using solar power and some of the recovered mass as propellant
Some of the technologies needed to avert comet or asteroid impact are similar to those needed to recover the diverse resources contained in these bodies. Thus it is desirable to develop this technology, and asteroidal resources, both to achieve space industrialization, species security, and long term prosperity, and to build the capacity to avert disaster.
This paper reviews concepts for mining the Near-Earth Asteroids for supply of resources to future in-space industrial activities. It identifies Expectation Net Present Value as the appropriate measure for determining the technical and economic feasibility of a hypothetical asteroid mining venture, just as it is the appropriate measure for the feasibility of a proposed terrestrial mining venture. In turn, ENPV can obviously be used as a ‘design driver’ to sieve the alternative options, in selection oftar get, mission profile, mining and processing methods, propulsion for return trajectory, and earth-capture mechanism
POPULATION OF TARGET BODIES:
Recent progress in asteroid search programs (especially LINEAR, www.ll.mit.edu/LINEAR/)has been spectacular. According to Dr David Morrison, in the latest issue of his NEO Newsletter (dmorrison@arc.nasa.gov), there are now about 1400 identified NEOs, defined as objects whose orbit shave a perihelion less than or equal to 1.3 AU. Of these, about 300 are classified as PHAs (Potentially Hazardous Asteroids), meaning that their orbits come within 0.05 AU (7.5 million km) of the Earth’s. Of the total 1400 known NEOs, some 500 are of absolute visual magnitude less than 18, and therefore nominally of diameter larger than 1 kilometre. This recently derived number is larger than had previously been considered likely
Estimates based on the serendipitous rediscovery rate indicate that the total population of “>1 km bodies” within the NEAs is now at least 1000, and the total population of “>100 metre bodies” is now thought to be in the range 100,000 to 200,000 (Lewis, 1993)1Logistical Implications of Water Extraction from Near-Earth Asteroids, SSI Princeton Conference
Assessments originally performed by Lau and Hulkower (1985)2, and later by Lewis (1993),indicated that something like 10% of all NEAs are more accessible, energetically speaking, than the Moon, and are very much easier to return from, than the Moon. These estimates were recently reviewed by the present author, using the Shoemaker-Helin formulae for estimating the probable likely minimum delta-v for Hohmann transfers to and from these bodies (Helin & Shoemaker 1978)3, and show that we can now identify about 90 specific named bodies which are more accessible than the Moon (viz, have a minimum out bound delta-v from LEO for rendezvous of less than 6 km/s). About 200 have ‘global minimum’ out bound delta-v’s from LEO under about 6.5 km/s. A (very) few have outbound d-v’s under 4.5 km/s.(See Tables attached as Appendix) Similarly, a few have d-v’s for return departure of the order of 1 km/s.
Since the Tunguska explosion, now believed to have been caused by atmospheric breakup of a 60 meter diameter comet remnant (Report of the UK Task Force on Near Earth Objects, 2000), deliveredabout 20 Megatons of explosive energy, and given the likelihood that such impacts are more frequent than was first considered, (CCNet :http://abob.libs.uga.edu/bobk/cccmenu.html)it can be seen that there is indeed aserious need to attempt to find and track all of these bodies, including the many hundreds of thousands in the smaller size ranges, and indeed also to begin studies aimed at engineering deflection methods (Gehrels, 1994)4. Two comments should be made in passing: Firstly, the most likely scenario in which humanity will be faced with the necessity to ‘do something’ will be the discovery of a relatively small body, maybe a year or so prior to impact. The 10 km planet busters are rare, the ‘Tunguskas’ are much more likely. Secondly, much of the science and engineering relevant to working out how to deflect these bodies will also be directly relevant to working out how to extract resources from, and otherwise exploit them, and vice versa. For example, the main obvious alternative to nuclear explosive deflection would be to emplace a remote miner and rocket propulsion assembly on the threat body, which would extract and use the asteroidal material as reaction mass, to deflect the body’s trajectory. Thus, technologies for converting asteroidal mass into reaction mass will ‘enable’ both a highly controlled, ‘calibrated’, gentle deflection capability, and also mining and resource recovery. These propulsive methods might include solar thermal steam rocket, mass driver, or regolith rocket technologies
THE VARIETY AND COMPETITIVENESS OF ASTEROIDAL RESOURCES:
Near Earth Asteroids are of extremely variable and wide-ranging compositions, according to interpretations based on spectroscopic studies and on ‘ground truth’ from meteorites (Resources of Near-Earth Space, Lewis, Matthews and Guerrieri, University of Arizona Press, 1993)5. They include stony silicates, with enhanced levels of semiconductors and of Platinum Group Metals (Gaffey & McCord, 19766;Kuck, 1979)7; bituminous or carbonaceous material-bearing bodies (e.g., Zuppero, 1996)8; dormant orextinct comets with remnant ices and clay minerals; and reduced metallic bodies, composed in large part o
1. Lewis JS: “Logistical Implications of Water Extraction from Near-Earth Asteroids”, Space Studies Institute Princeton Conference, 1993.
2. Lau and Hulkower: “On the Accessibility of Near-Earth Asteroids”, AAS-AIAA Astrodynamics Conference, 1985.(paper AAS 85-352)
3. Helin & Shoemaker, “Earth Approaching Asteroids as Targets for Exploration”,in NASA Conference Publ.2053,Asteroids: an Exploration Assessment, ed Morrison and Wells, 1978.
4. Gehrels T:“Hazards Due to Comets and Asteroids”, University of Arizona Press, 1994
5. Lewis Matthews & Guerrieri: “Resources of Near-Earth Space”, University of Arizona Press, 1993.
6. Gaffey & McCord: “Asteroids: a Source of Natural Resources for Terrestrial and Extraterrestrial Applications”, Lunar Utilization Conference, 1976.
7. Kuck DL:“Near-Earth Extraterrestrial Resources”, in Space Manufacturing Facilities 3, AIAA, 1979
8. Zuppero A; “Discovery of Abundant Accessible Hydrocarbons Nearly Everywhere in the Solar System”,in Space 96, The Proceedings of the 5thInternational Conference on Engineering, Construction, and Operations in Space, American Society of Civil Engineers (ASCE)1996
Nickel-Iron alloy (e.g., 1986DA; see Ostro et al, 1991)9. All of these substances would be useful and valuable feedstock in the construction of infrastructure and supply of fuel for development of an orbital economy. Any industrial development in orbit requiring more than a few hundred tonnes per year of structural material or propellant will direct attention to these sources as ores, in the mining engineering sense.
This will happen because raw materials retrieved from asteroidal sources will not attract the high “airfreight” costs imposed by launch from Earth. The energy requirement to return material from many of the possible target near-earth asteroids is much less than that required to launch from Earth. In addition, the freedom to deliver the velocity change non-impulsively means that low power propulsion systems are acceptable, thus permitting a propulsion system that uses solar power and derives its return-journey propellant from the target body, such as asteroidal volatiles
Accessibility: In space, the parameter which determines how easy or difficult it is to deliver mass from one orbit to another, is not distance, but is the required velocity change,v, needed to perform the transfer. Likely lowestv targets for initial resource development are specifically the low eccentricity, low inclination subset of the “Earth-Approaching” Apollo, Amor, or A ten asteroids; or any as-yet undiscovered Earth-Trojan asteroid. Shortlists of high accessibility NEAs are attached as Excel files; their delta-vs are calculated using the Helin-Shoemaker estimation formulae.
The mission velocityv needed to reach selected “near earth” lower target asteroids is not much greater than that needed to place a communications satellite in geosynchronous orbit (GEO). Thevrequired to place material from these targets on an Earth-orbit-intercept trajectory may in selected cases be very much less than that required to lift mass into orbit from the surface of the earth, and can be imparted gradually, over several weeks, thus very substantially reducing the demands on the propulsion / power system.
Note at the outset that we must make the caveat that the delta-v requirement for the return trip is much more important than the d-v outbound, because the return trip places the most severe demand on the propulsion system
Target selection is important, because the lower the return propulsion requirement, the lower is the mass that has to be mined to produce propellant and therefore the lower may be the mining equipment mass, and the power supply mass, and the larger the proportion of recovered mass that can ultimately be sold for revenue.
It turns out that the major d-v requirement is for Earth-capture from heliocentric orbit into Highly Elliptical Earth Orbit, and then subsequently its reduction into LEO. Capture via powered or unpowered lunar flyby, followed by aerobraking (not aerocapture) will probably be part of the solution. This is discussed further below.
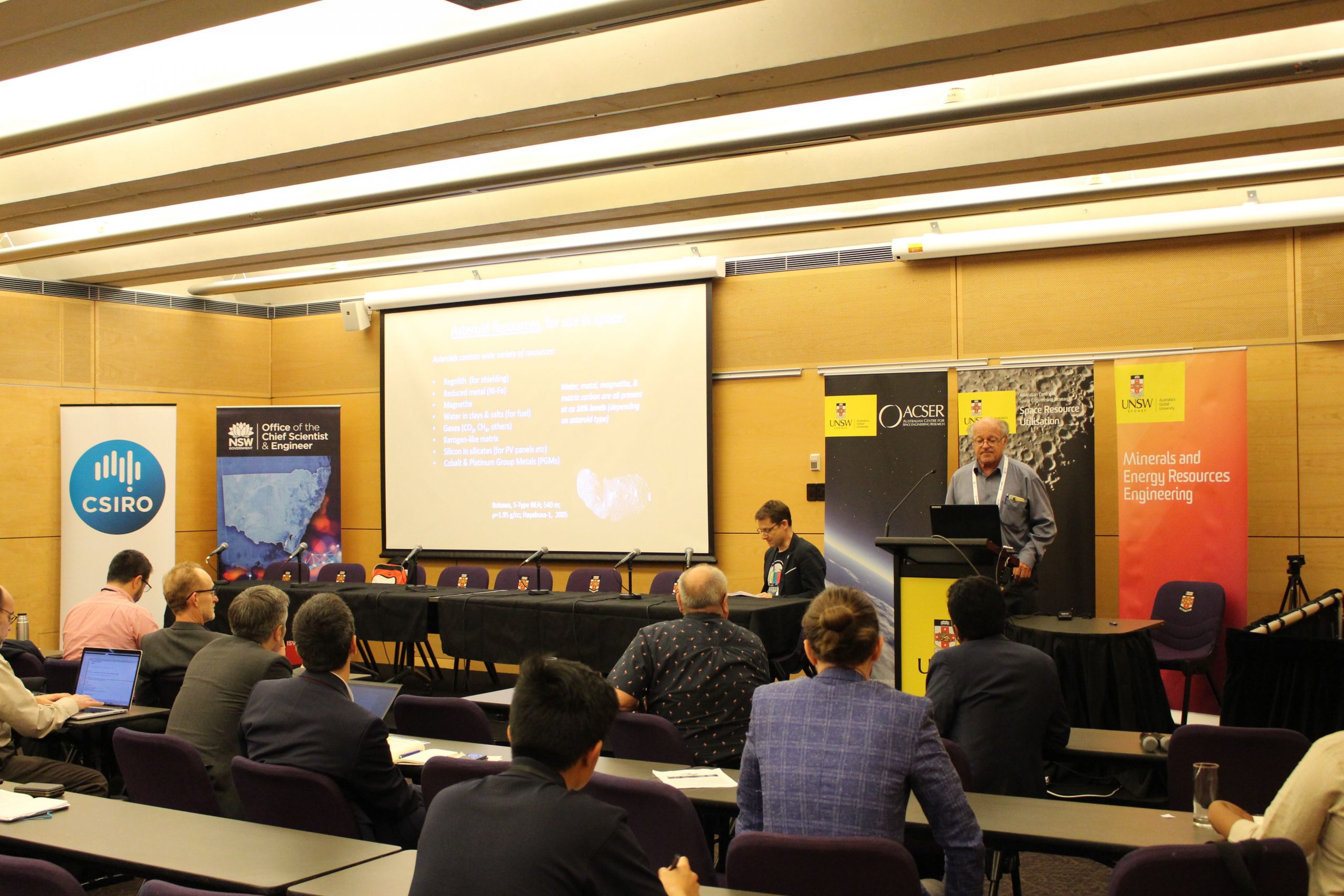
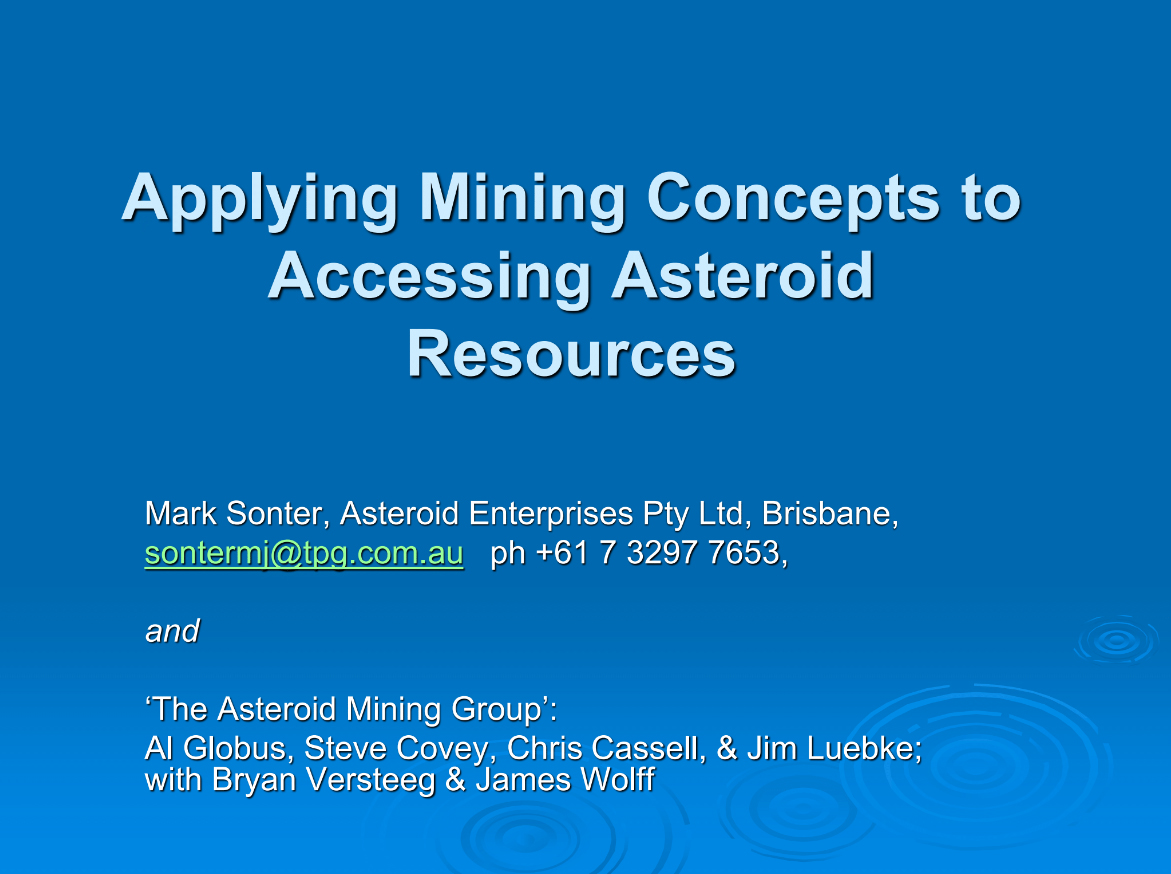
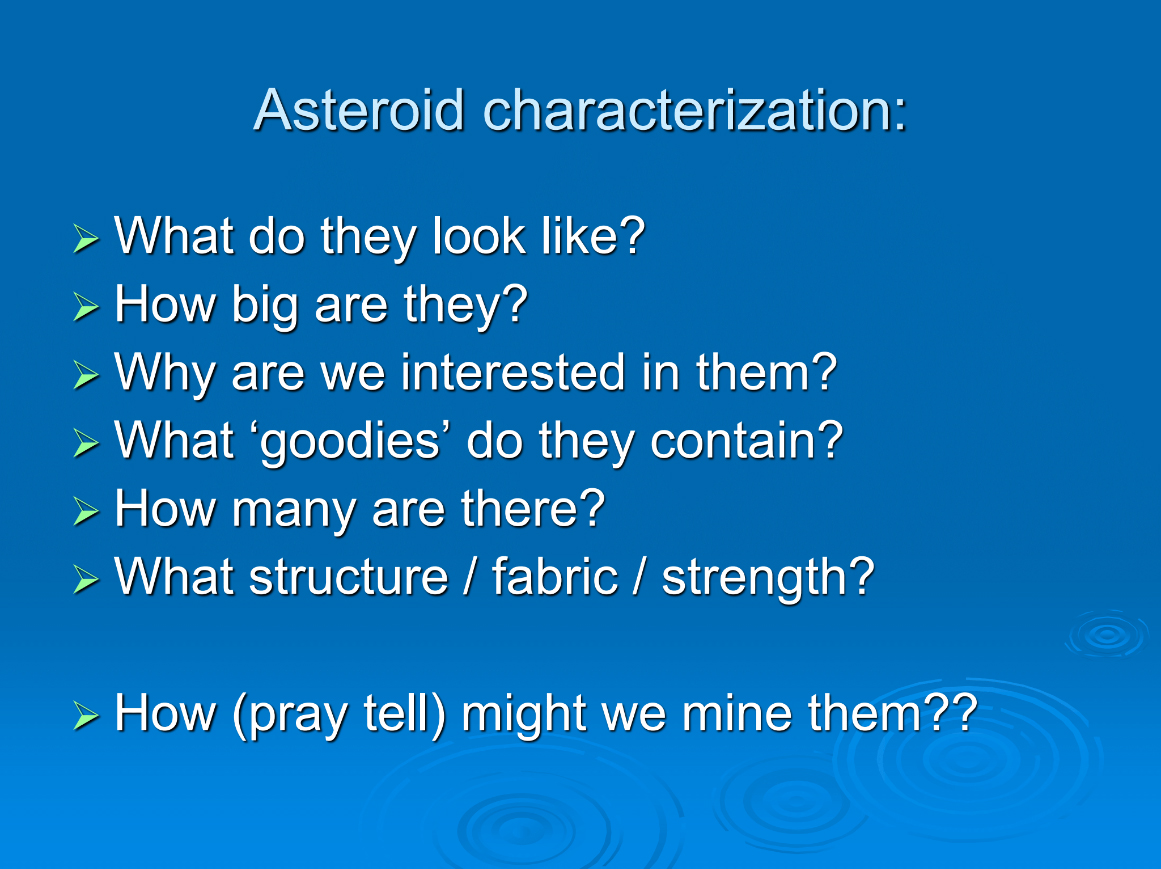
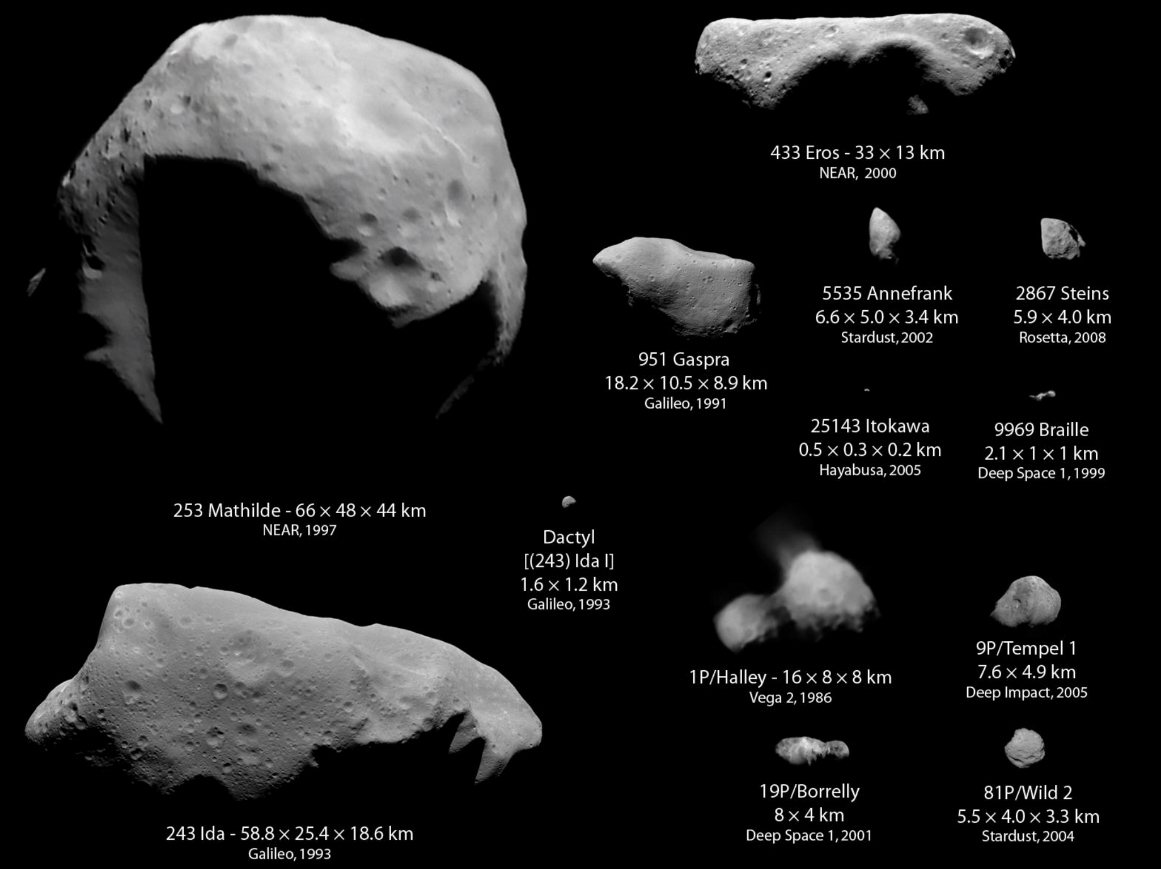
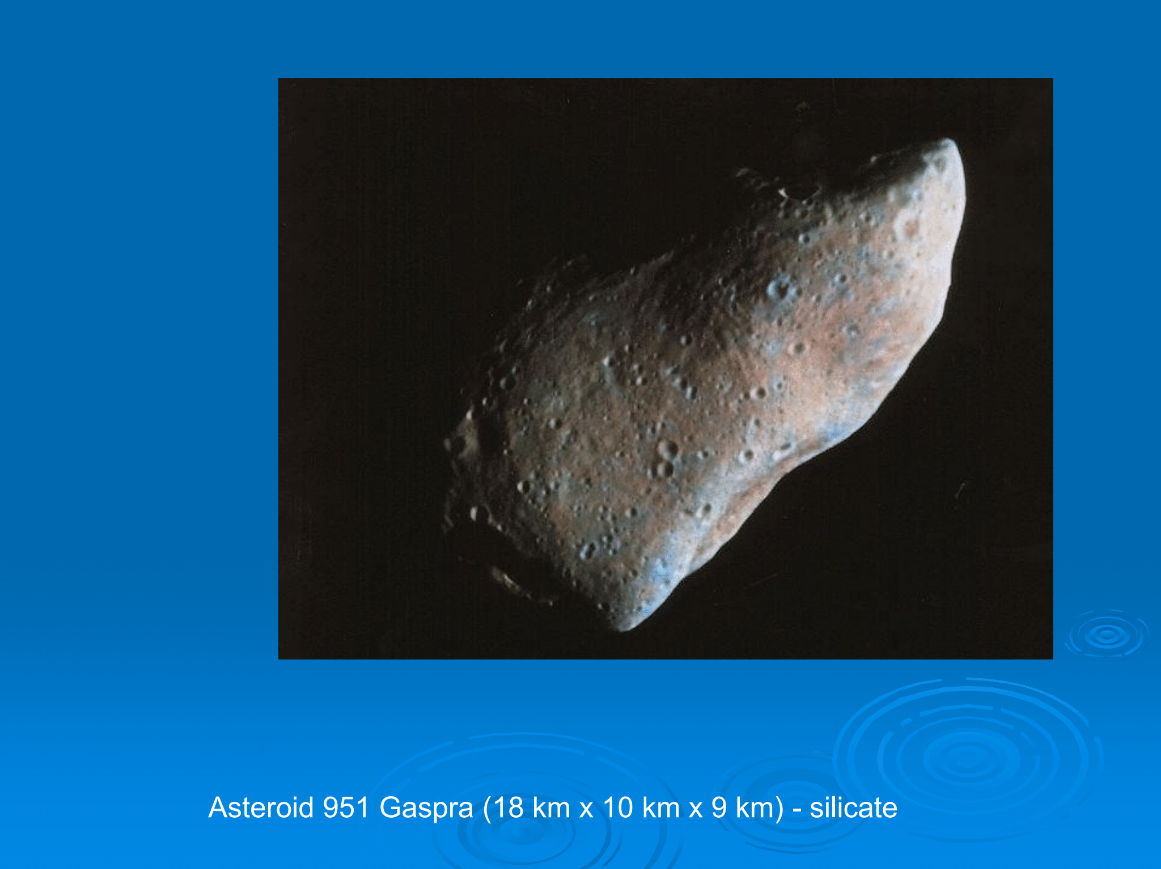
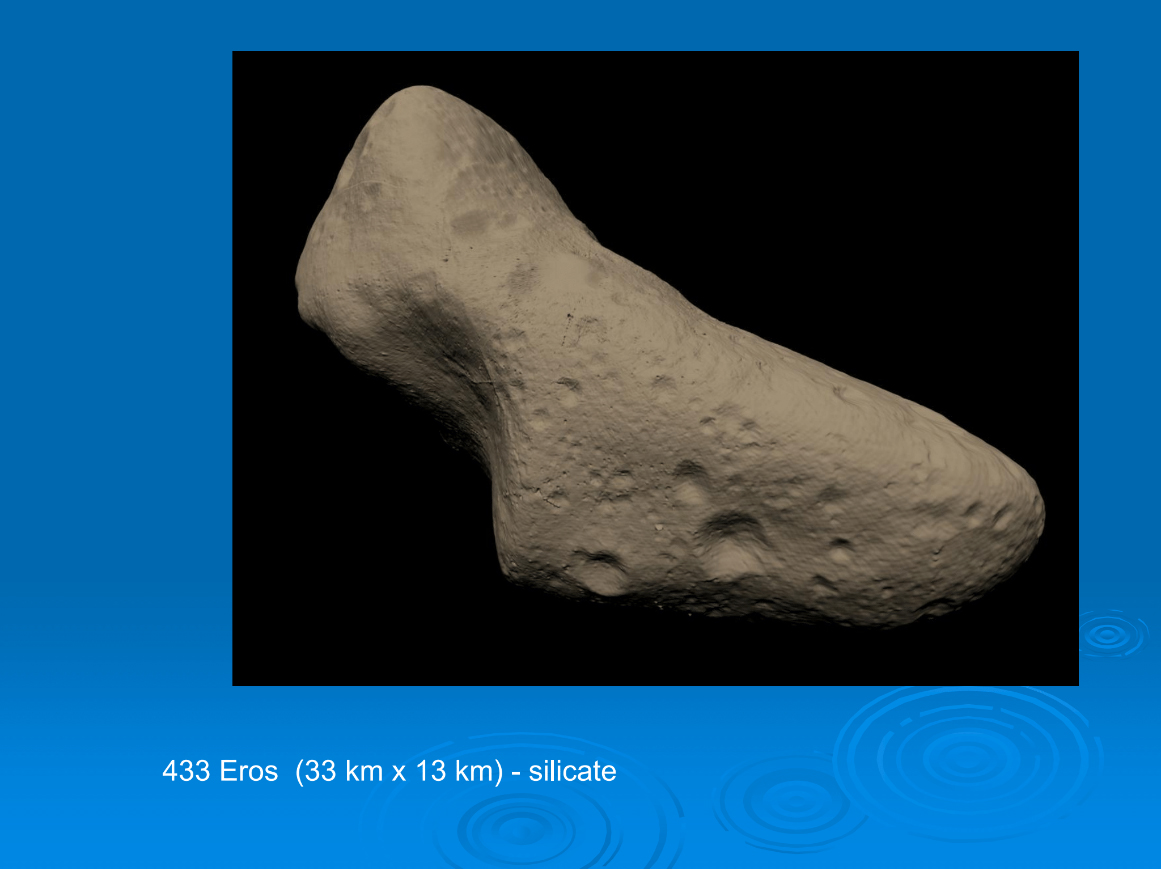
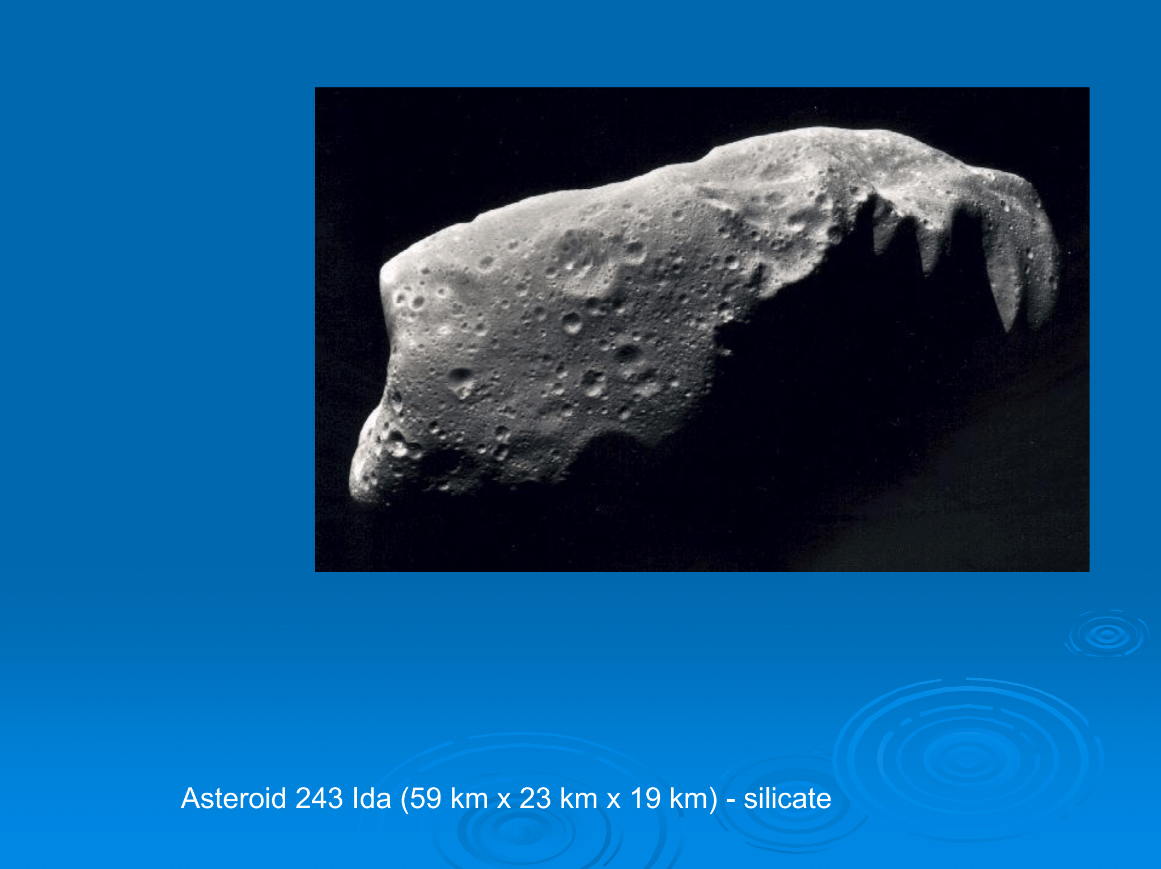
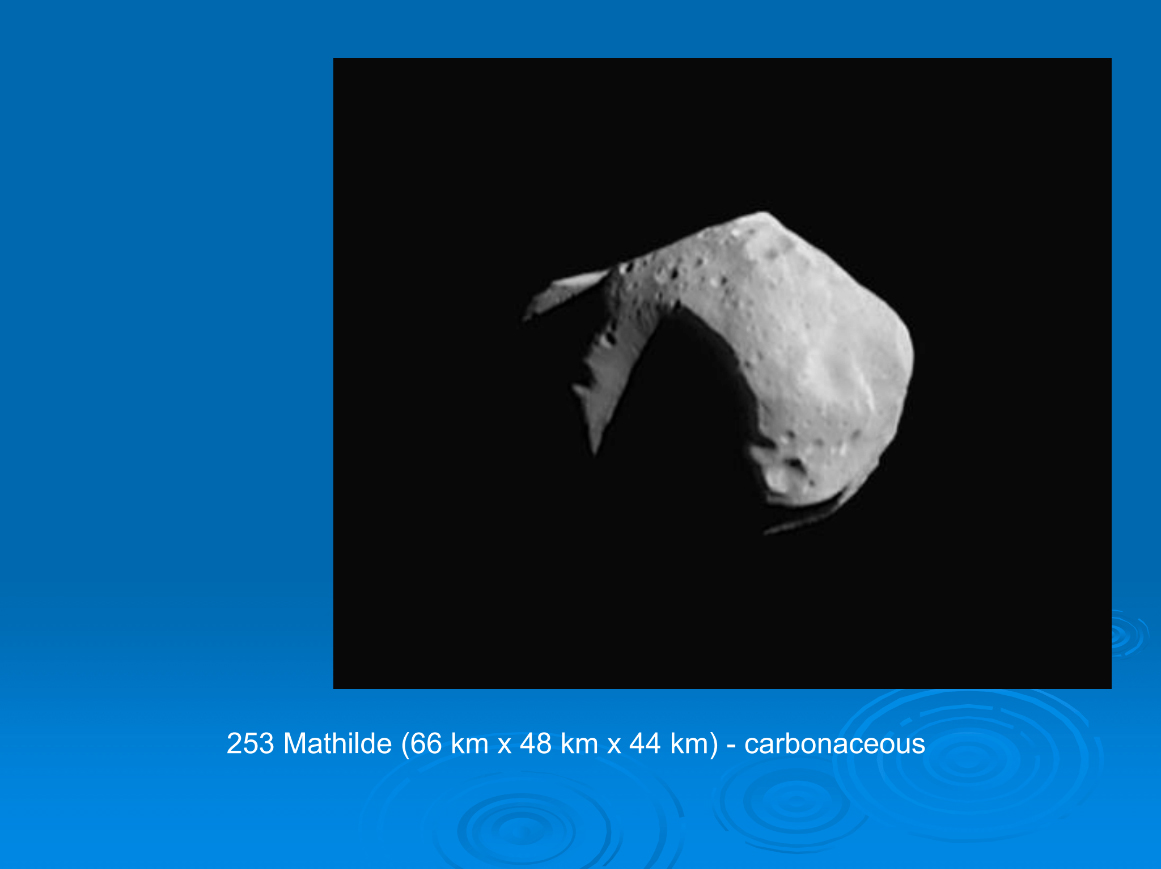
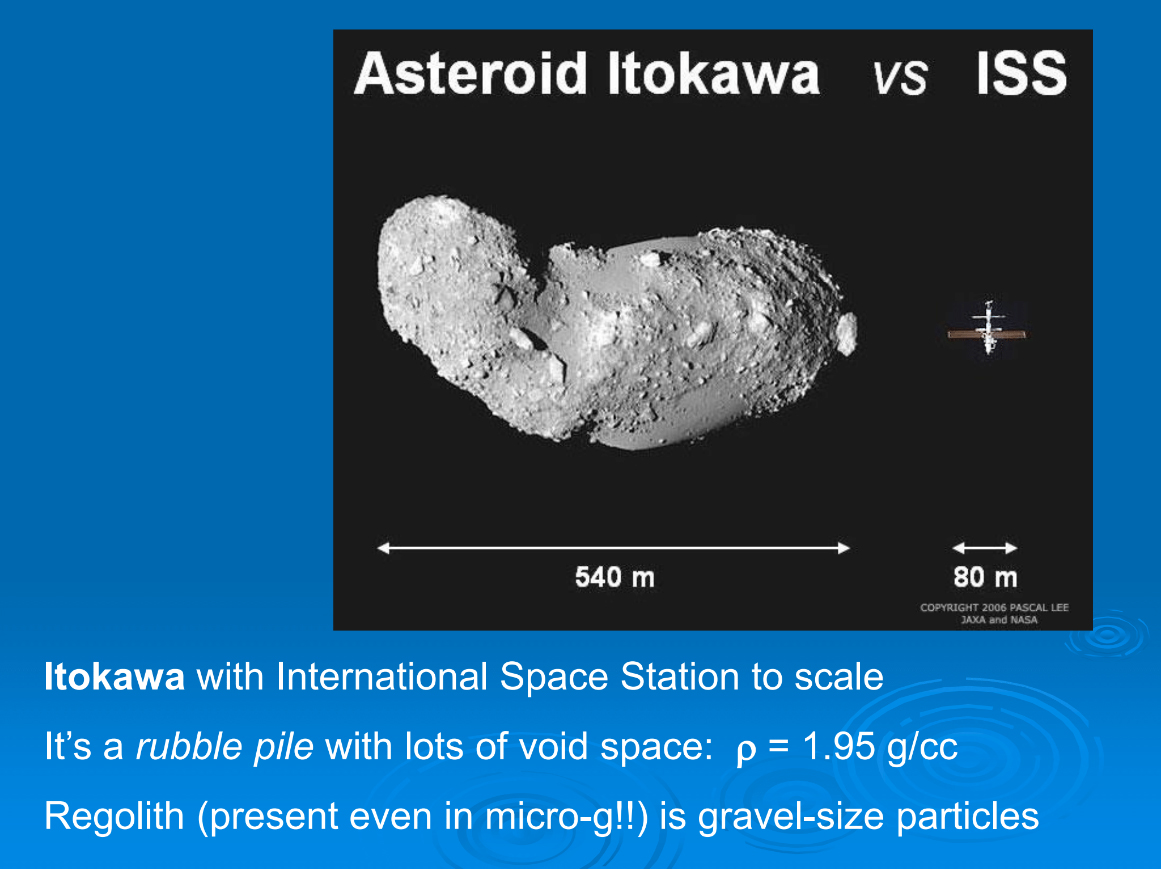
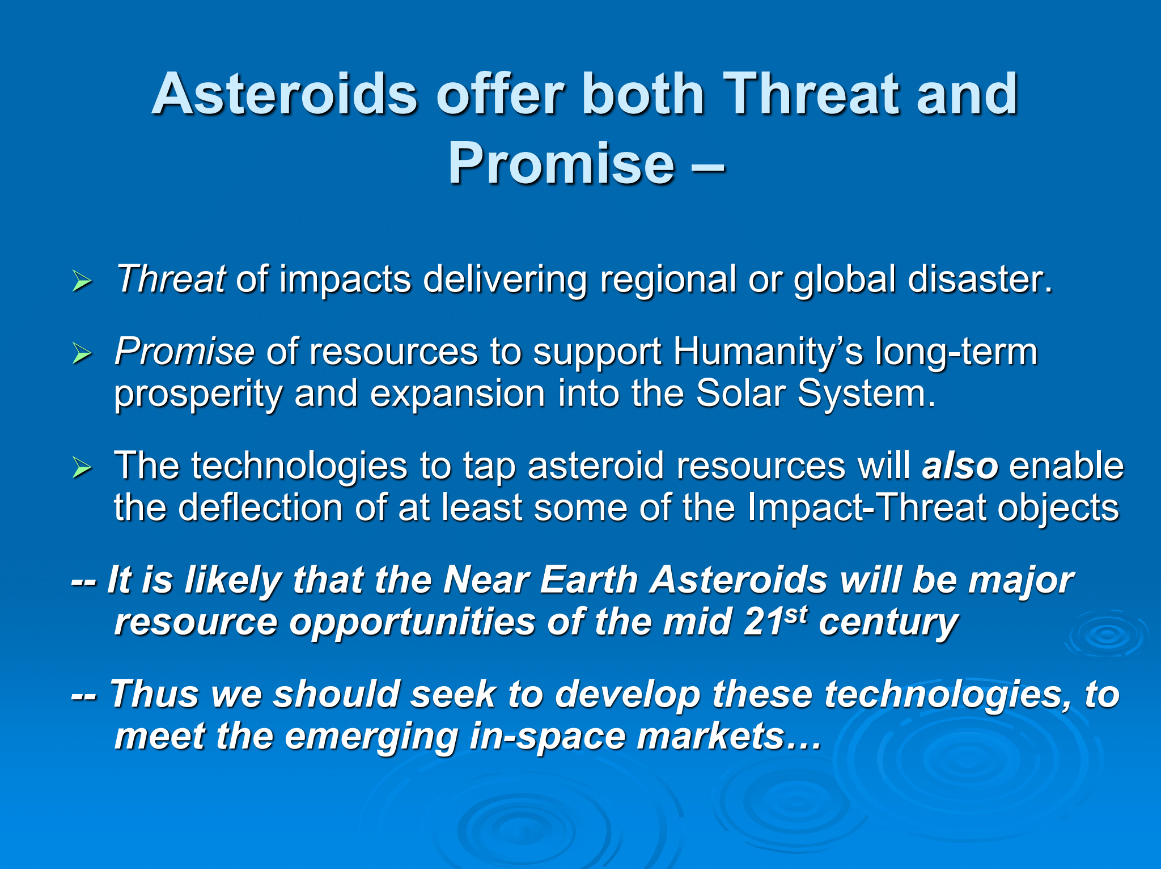
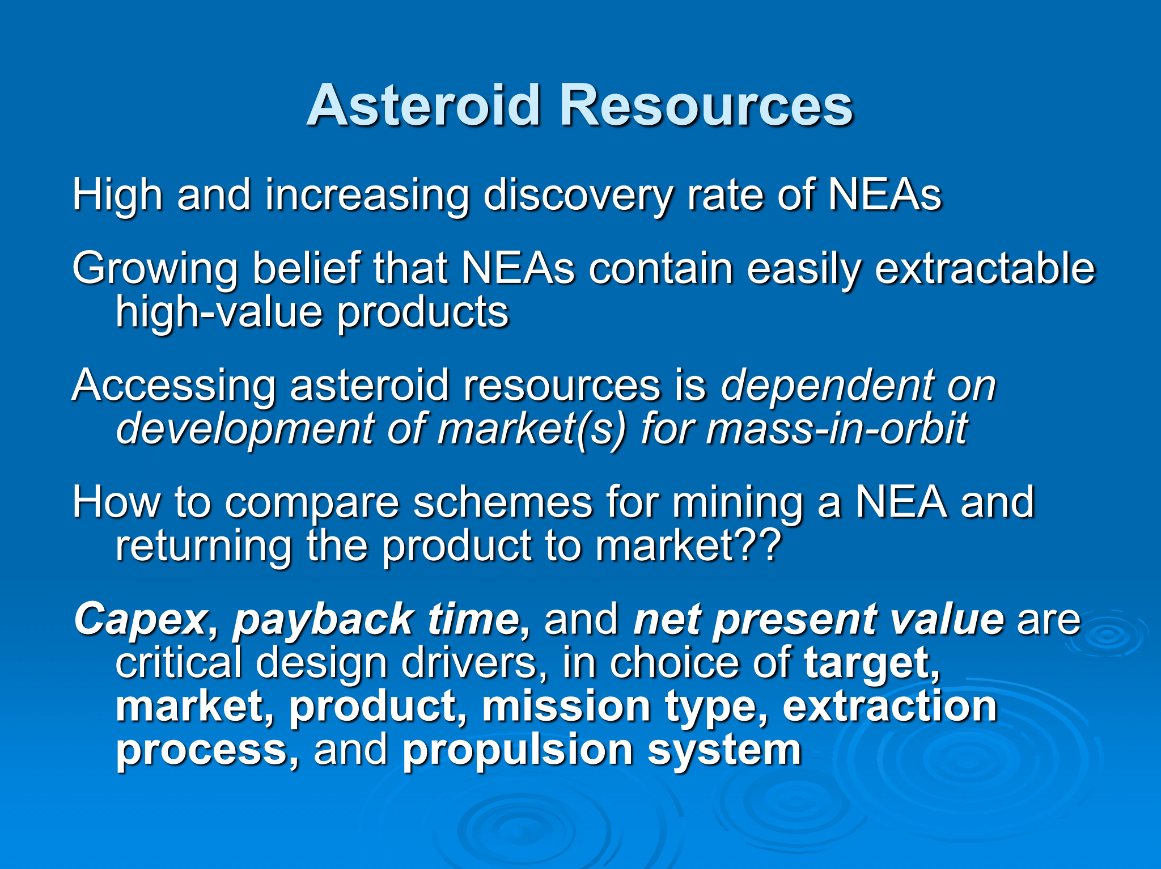
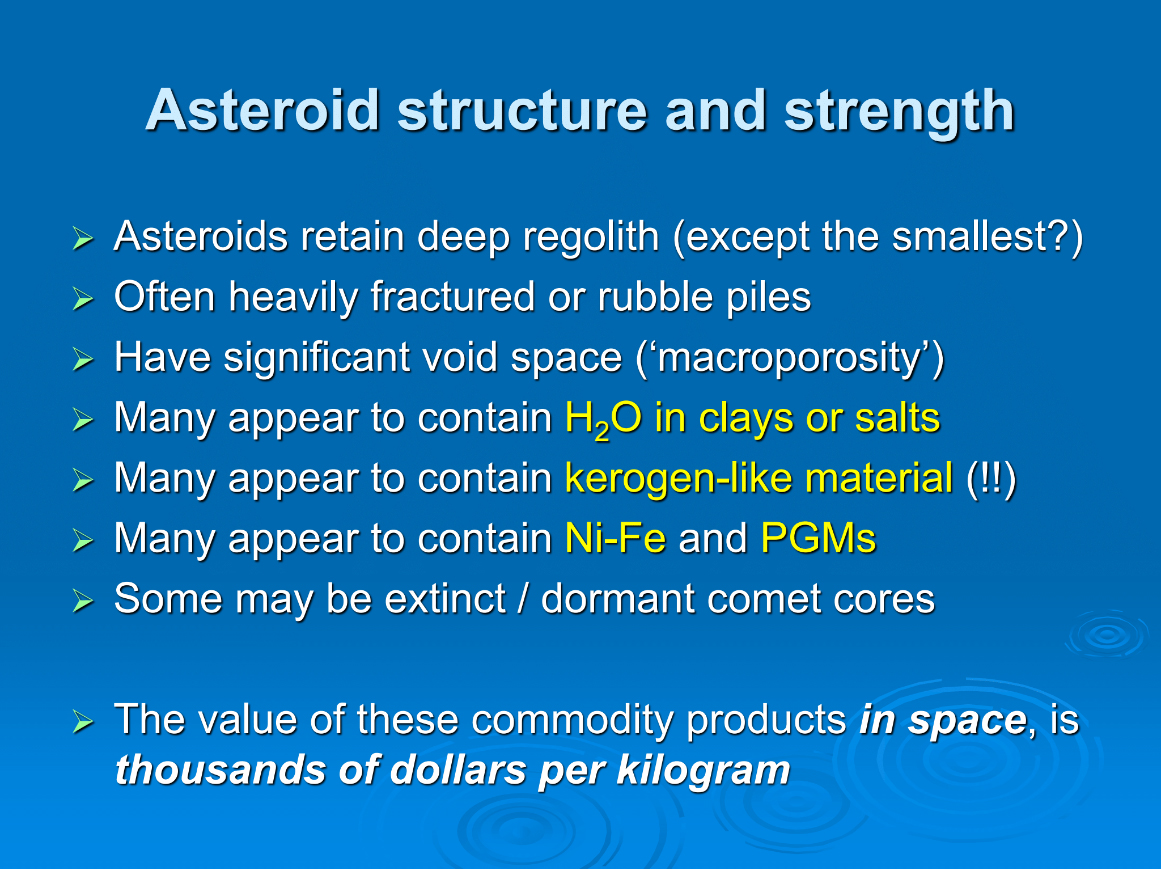
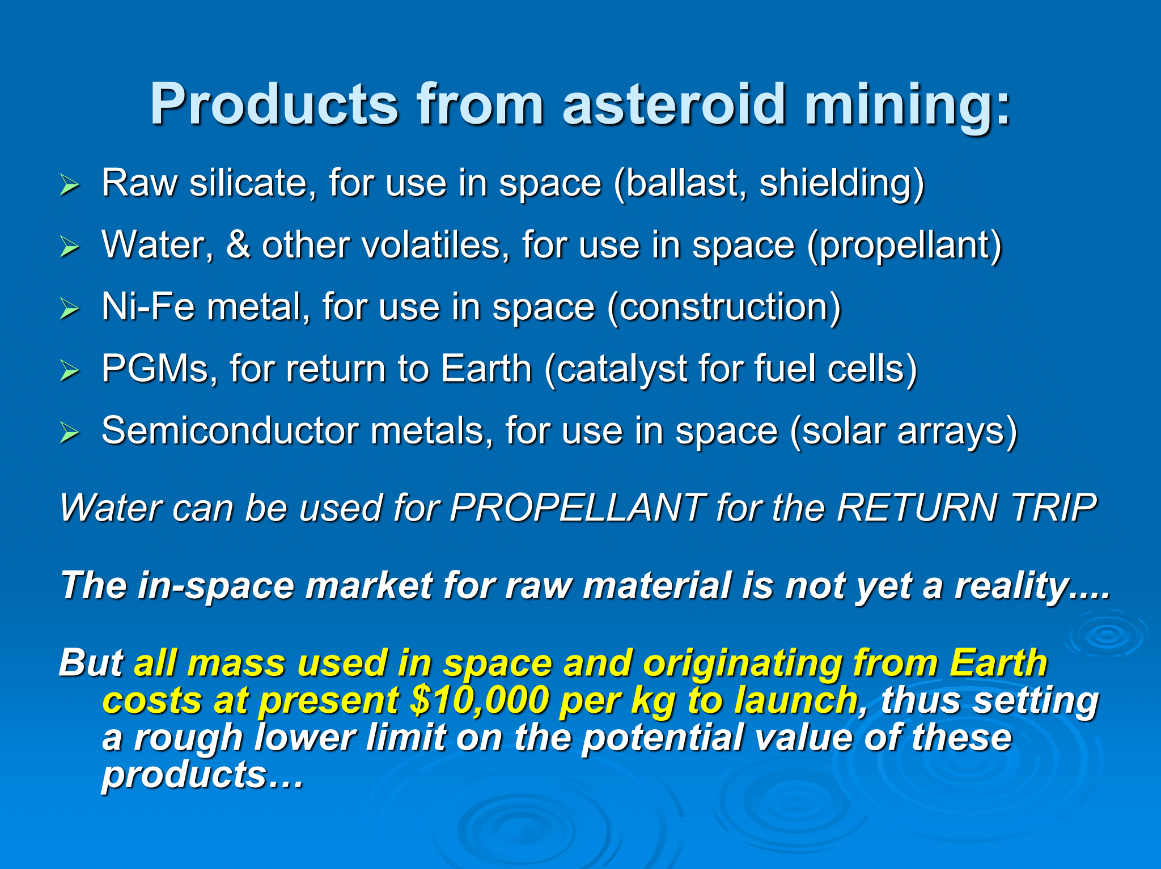
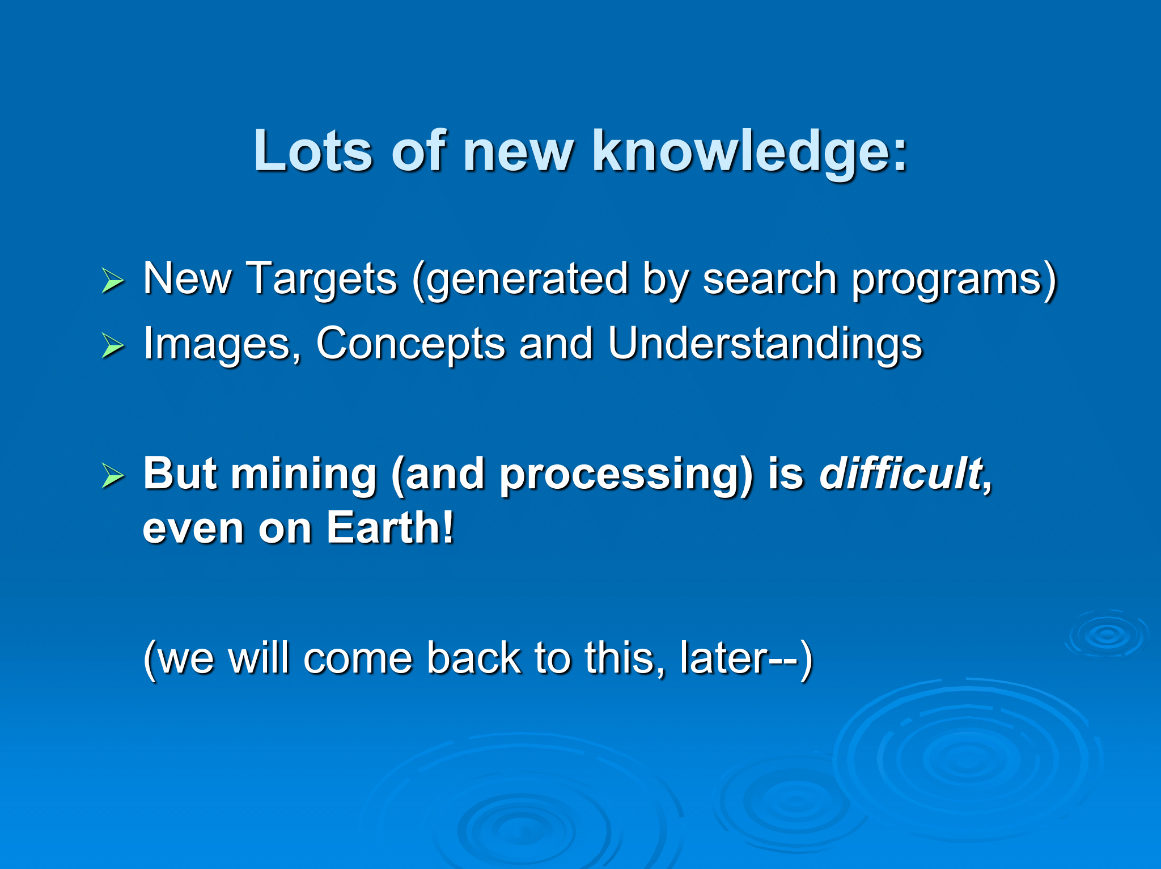
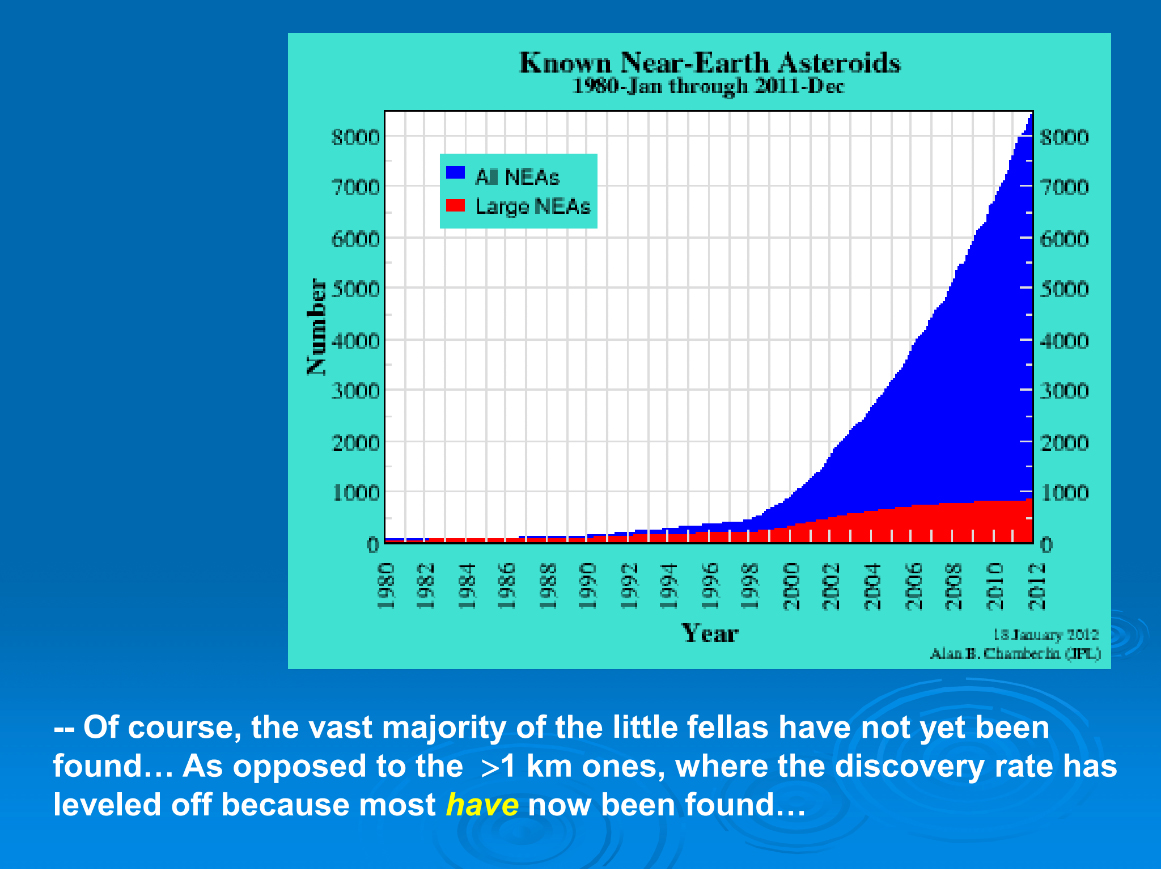
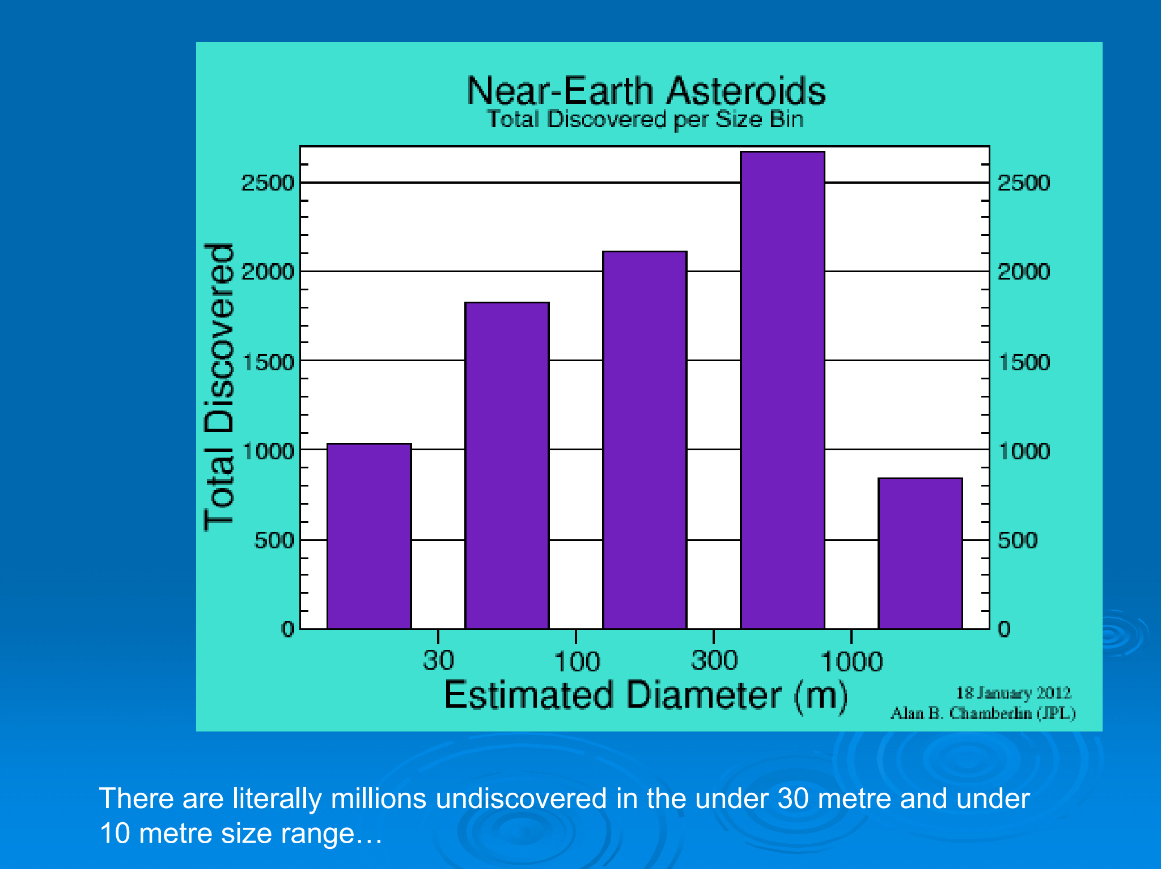
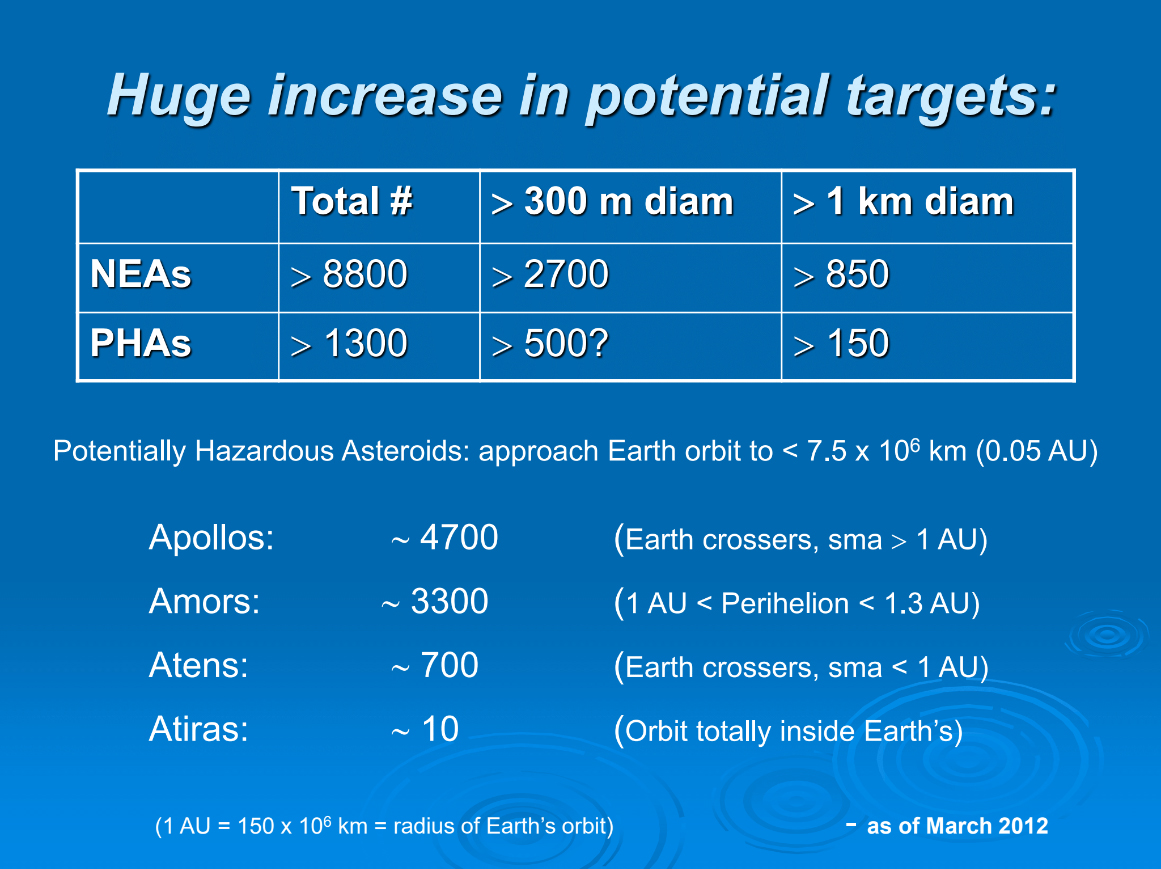
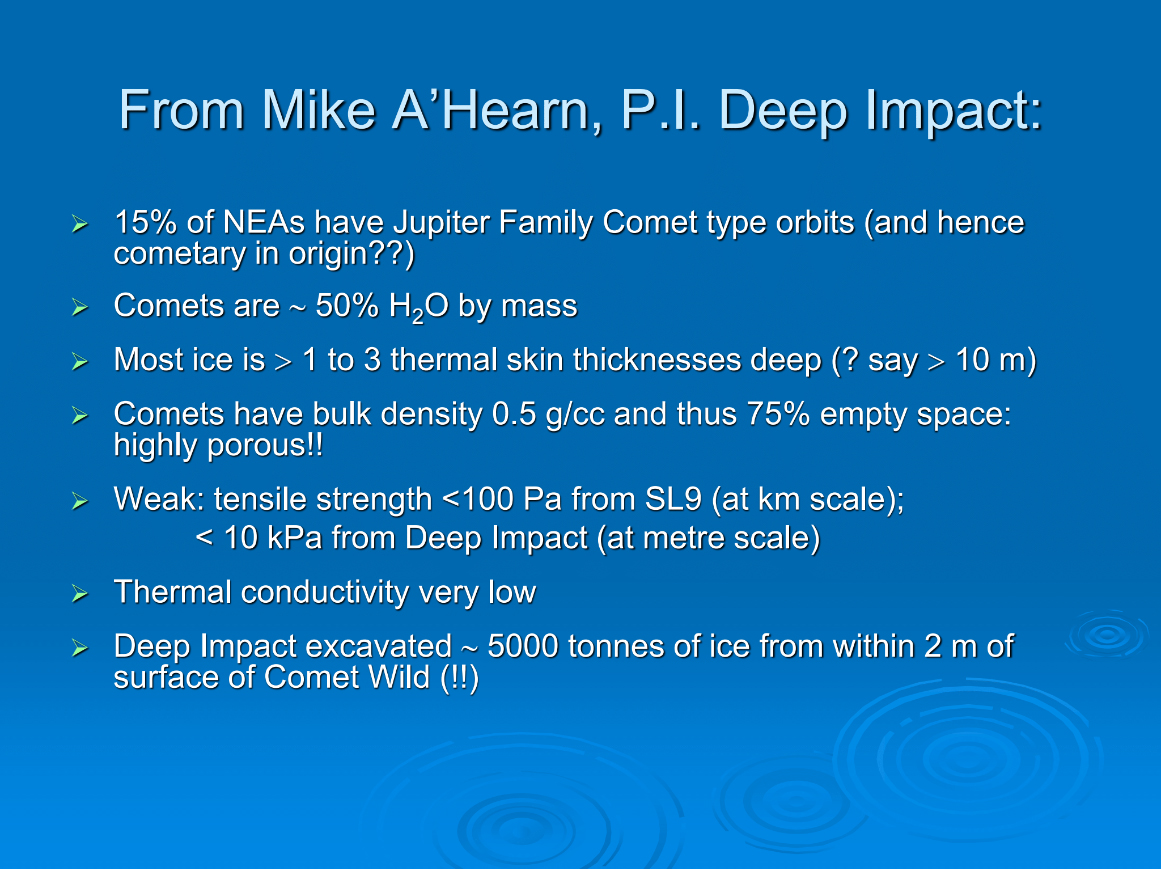
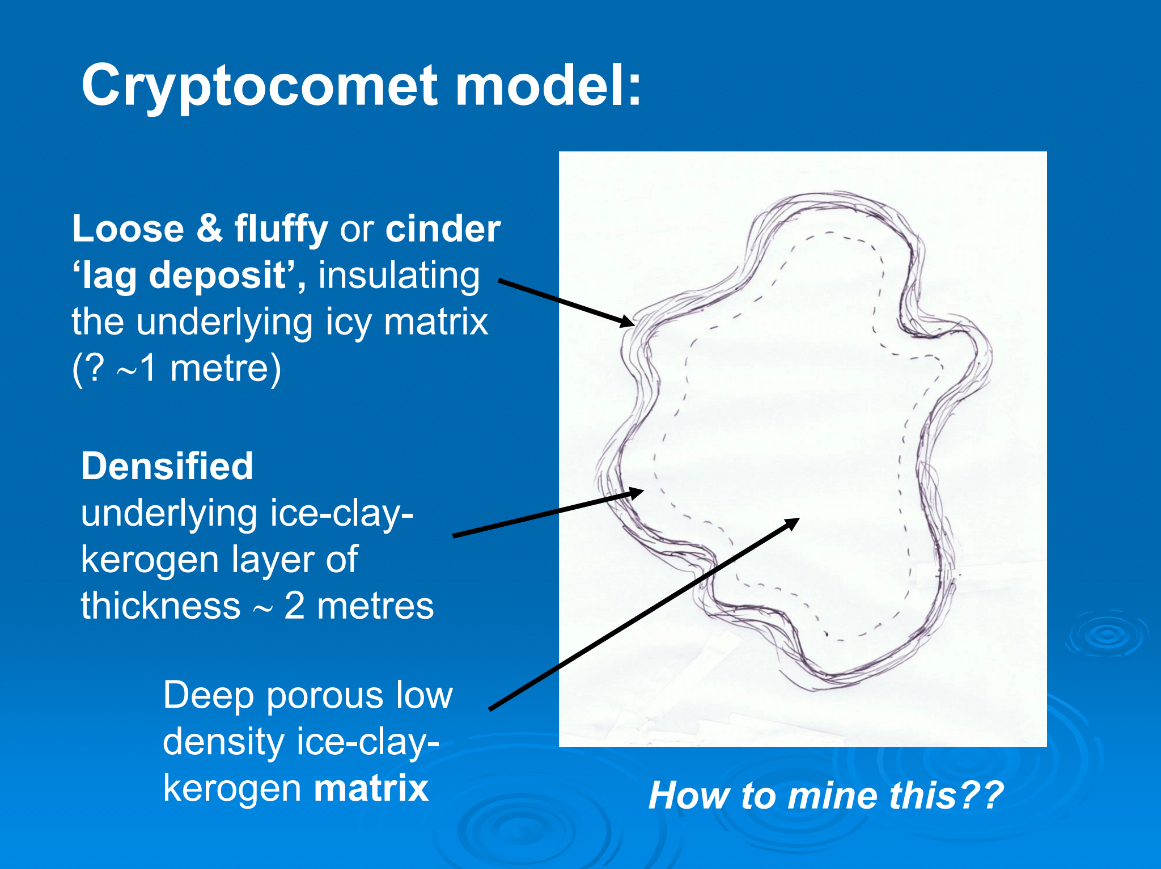
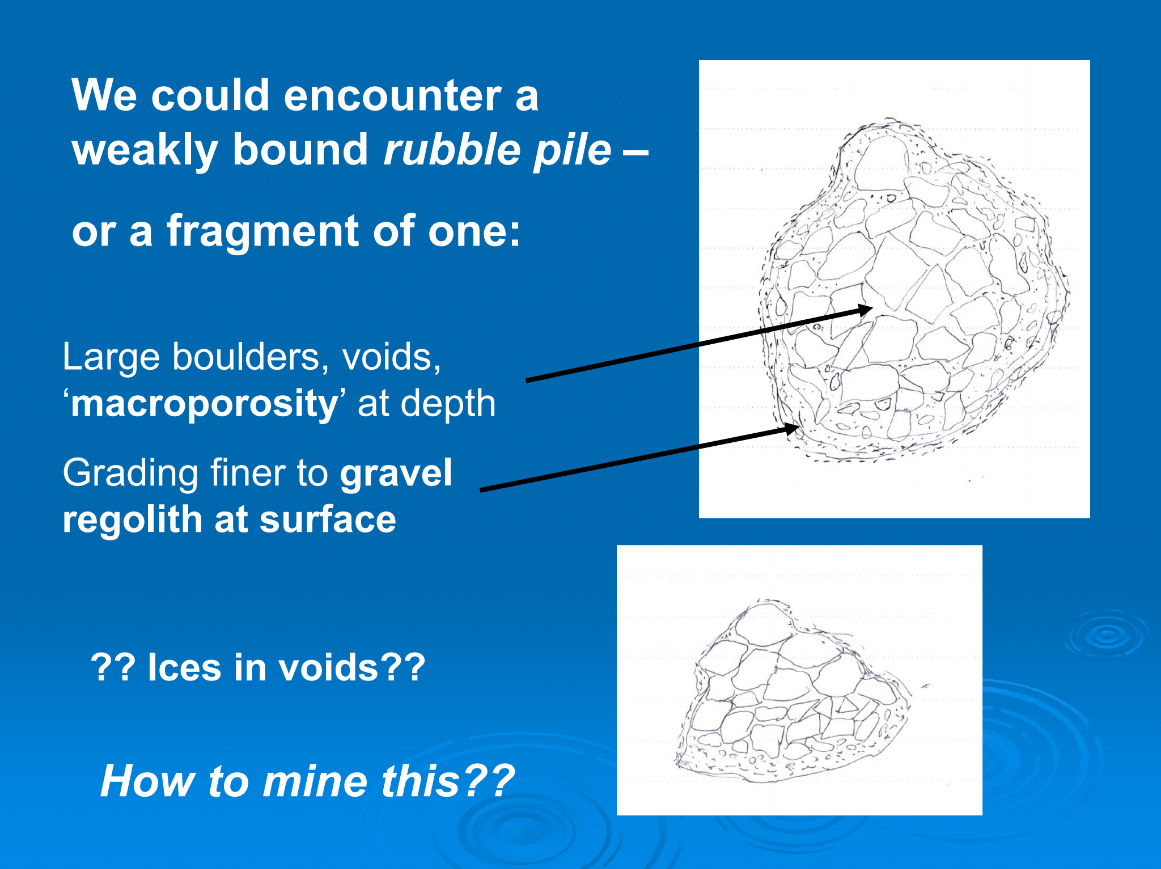
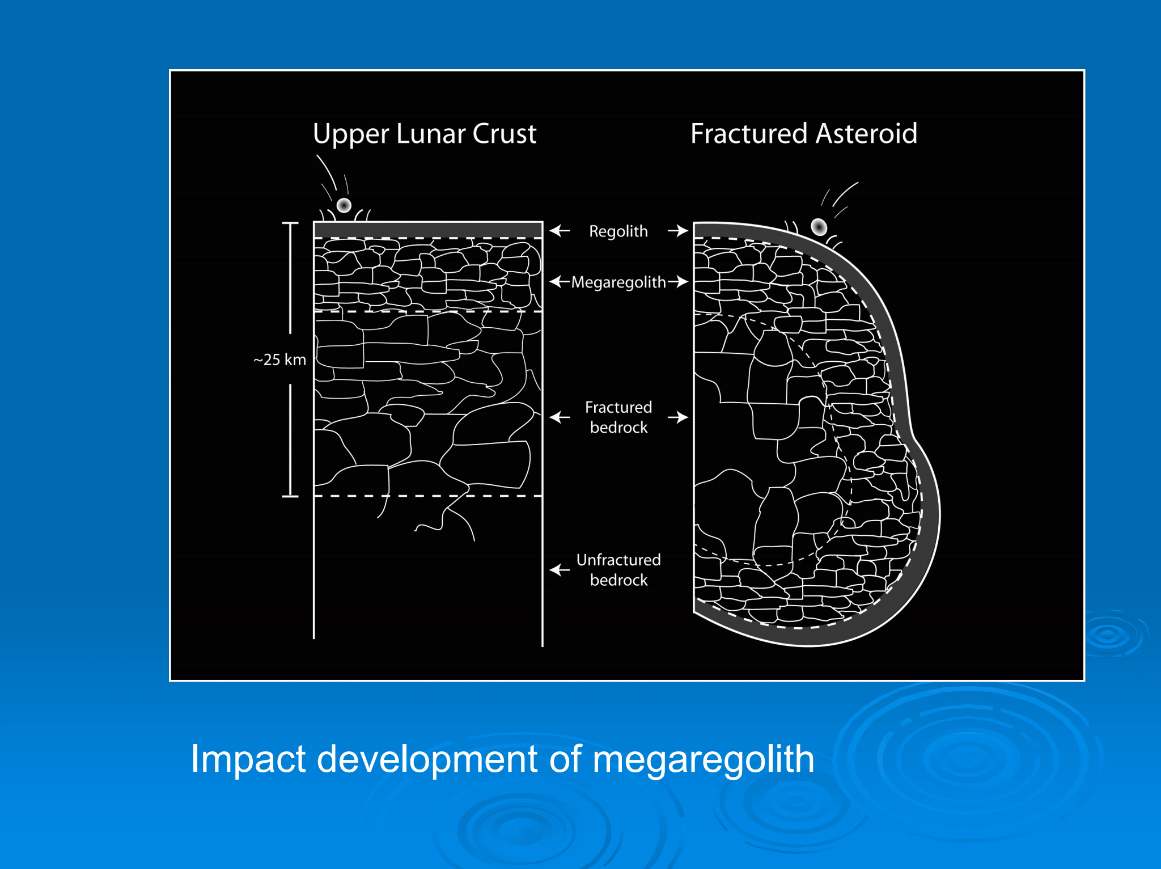
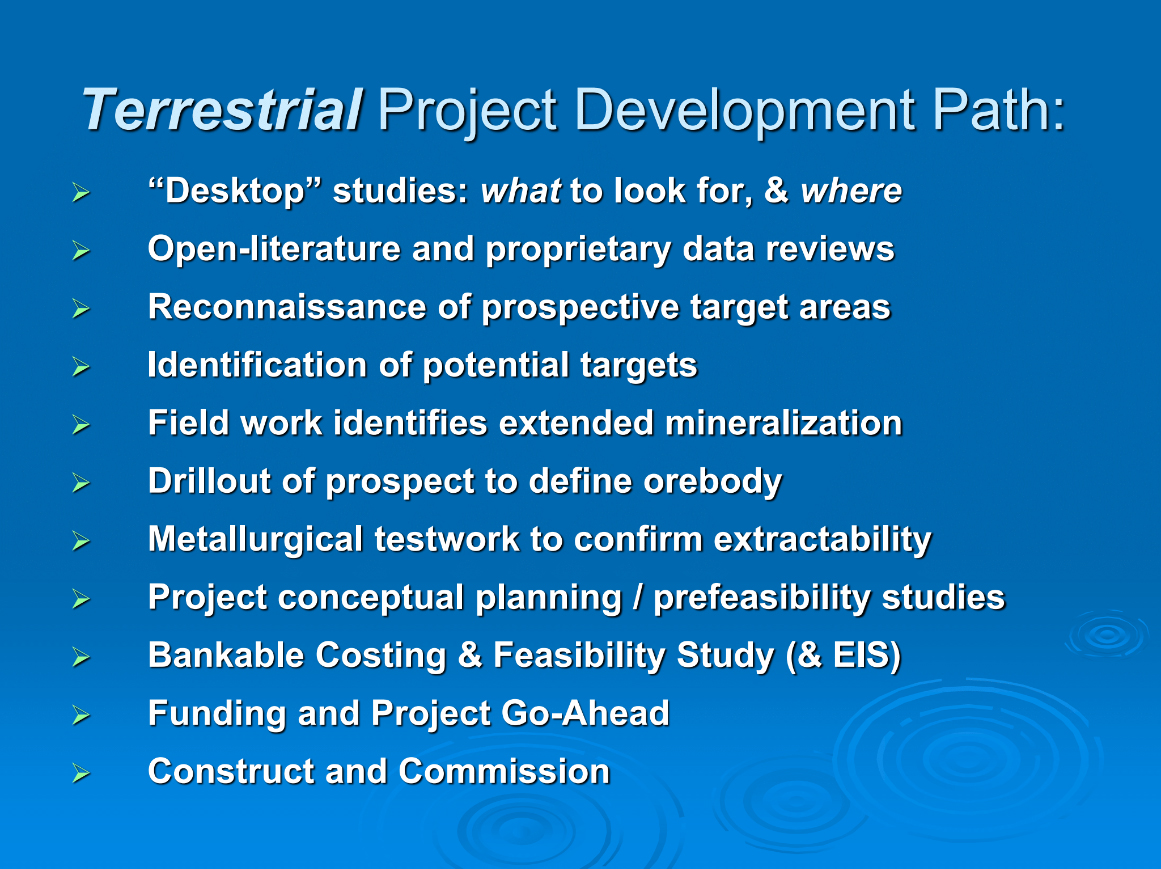
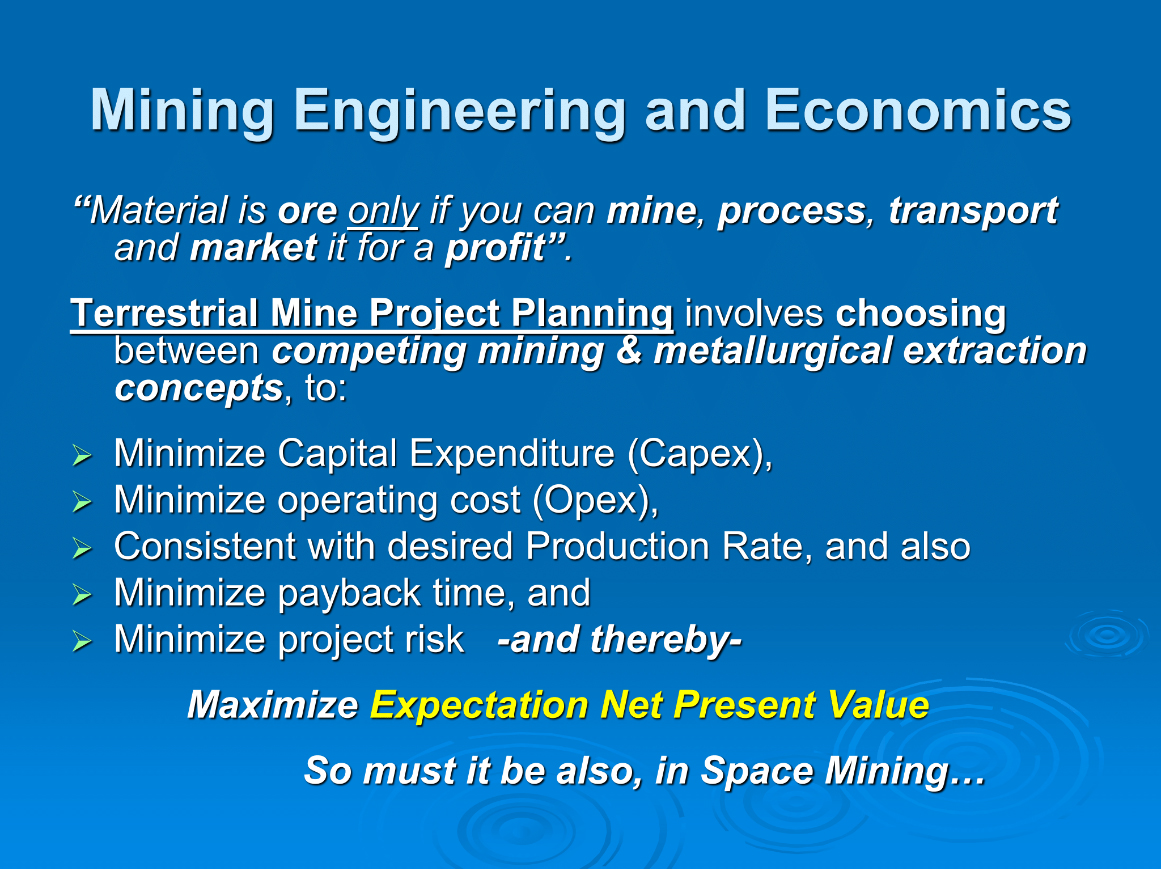
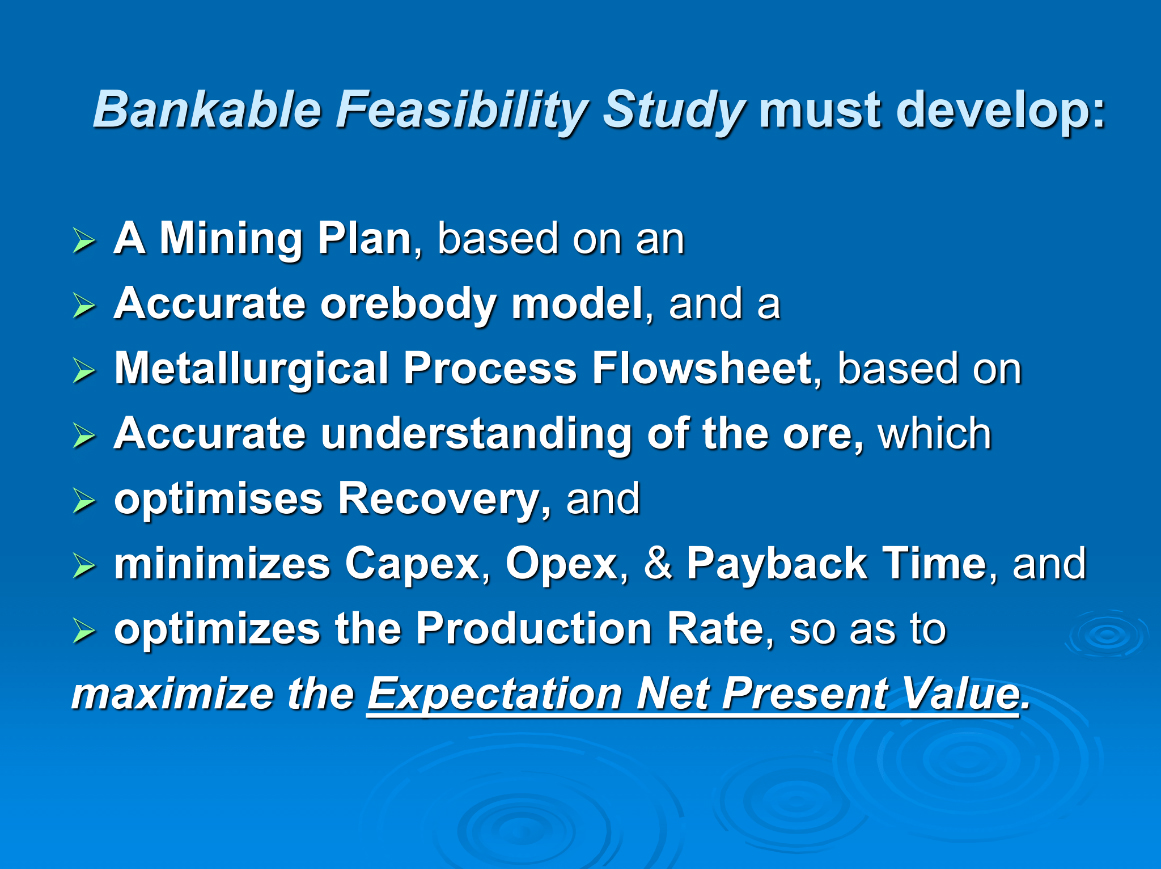
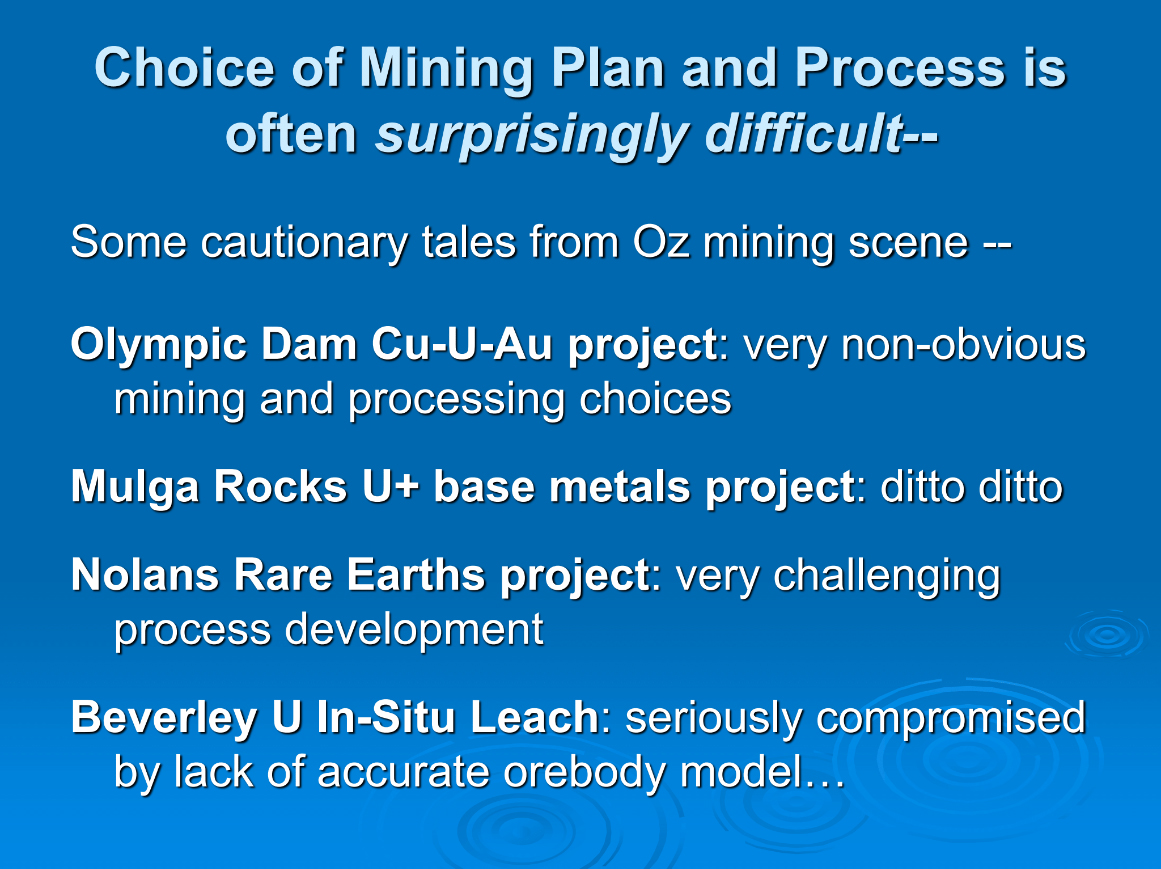
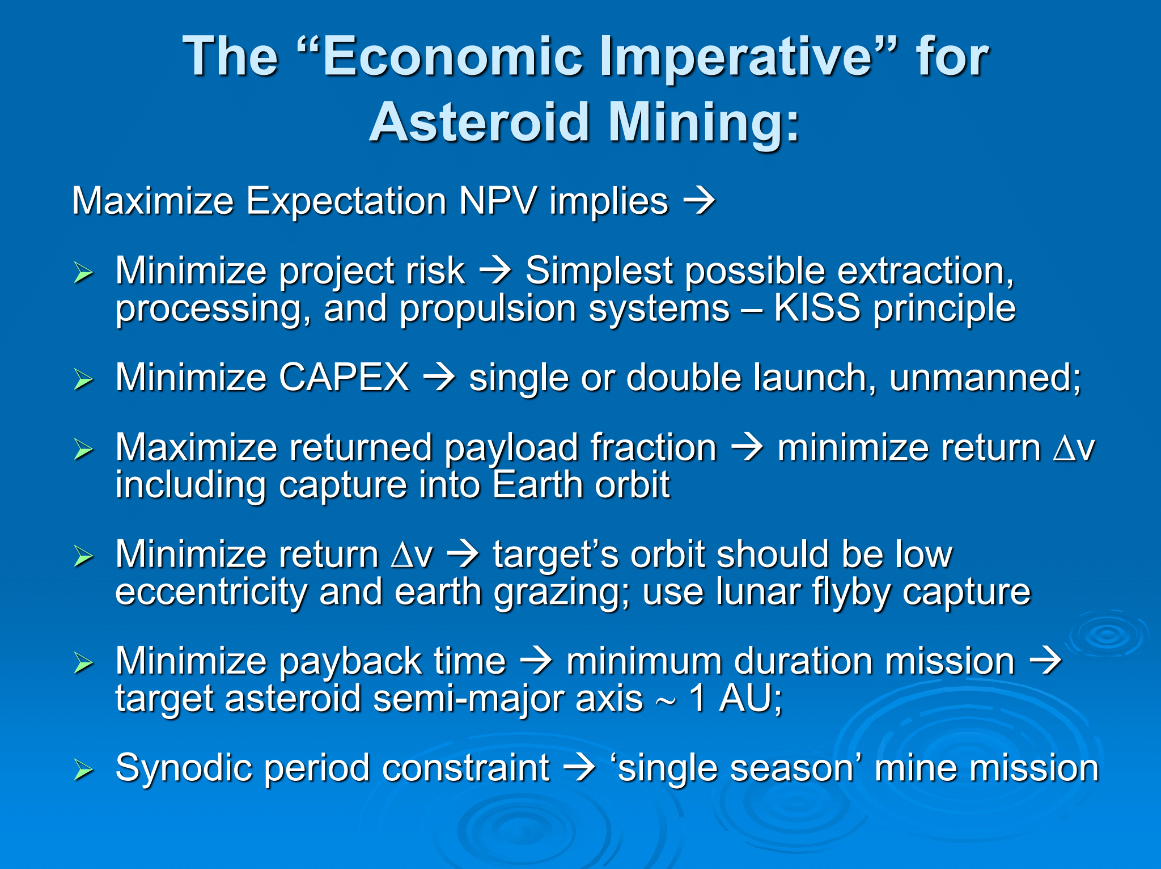
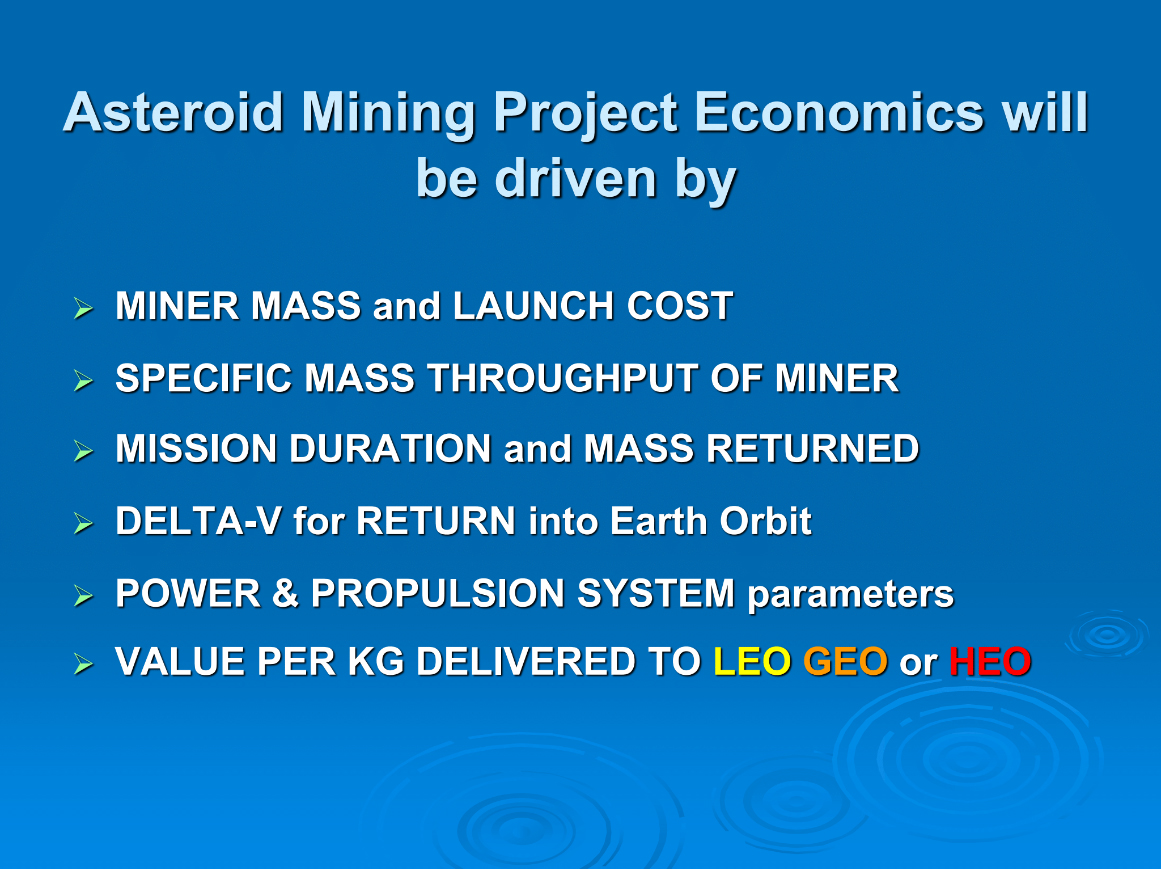
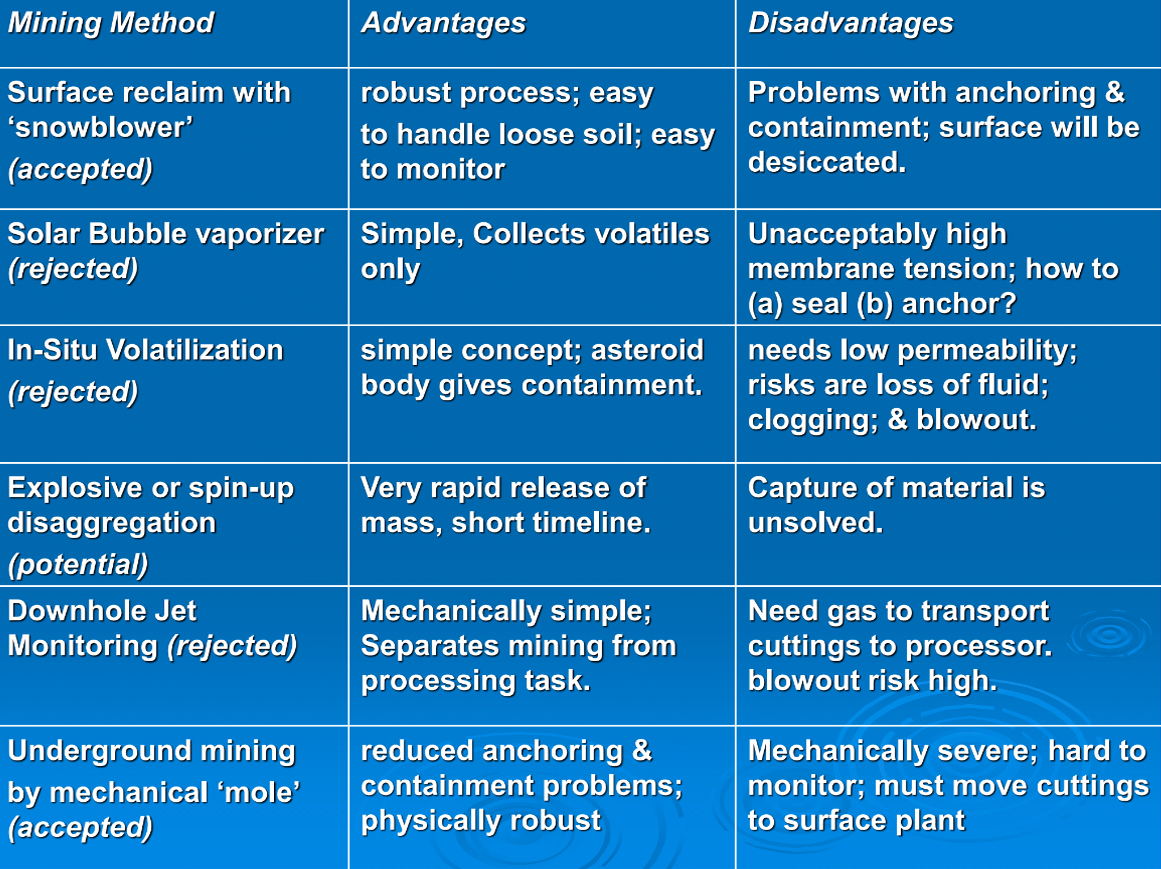
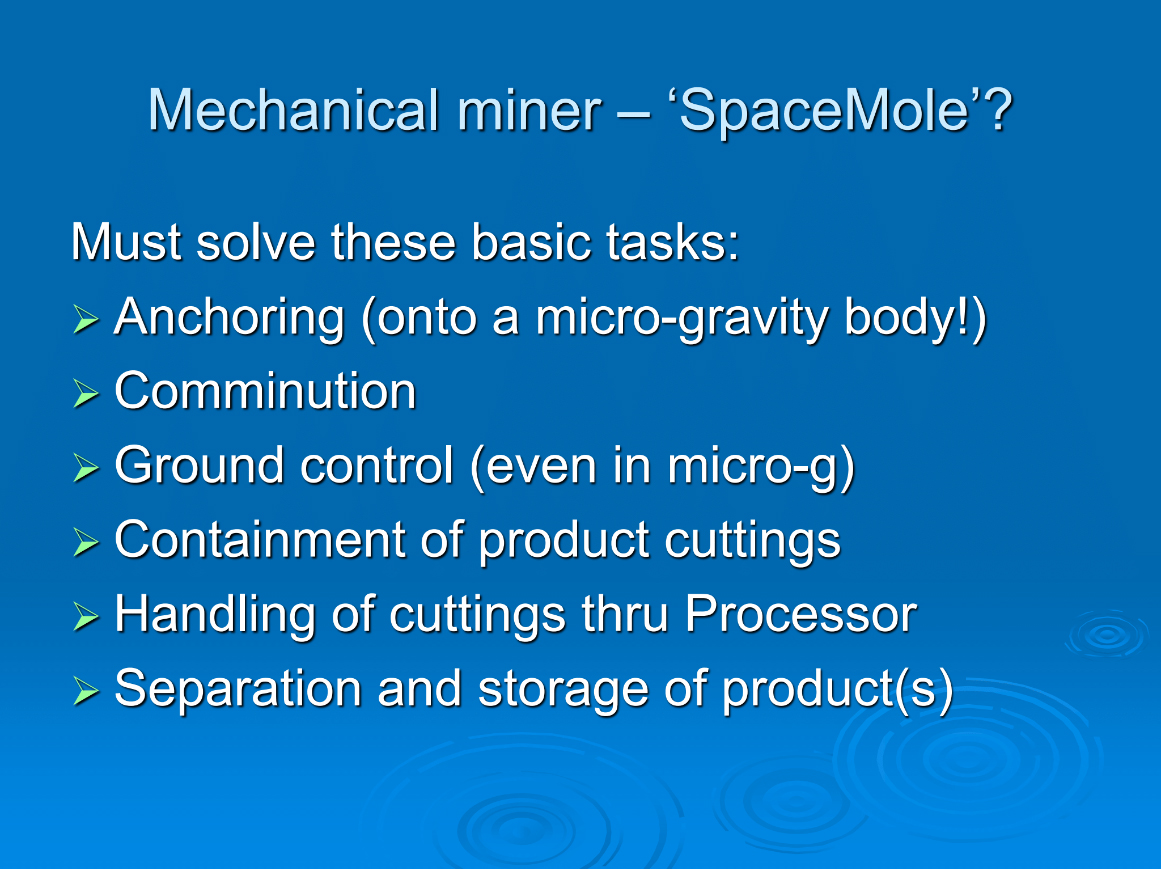
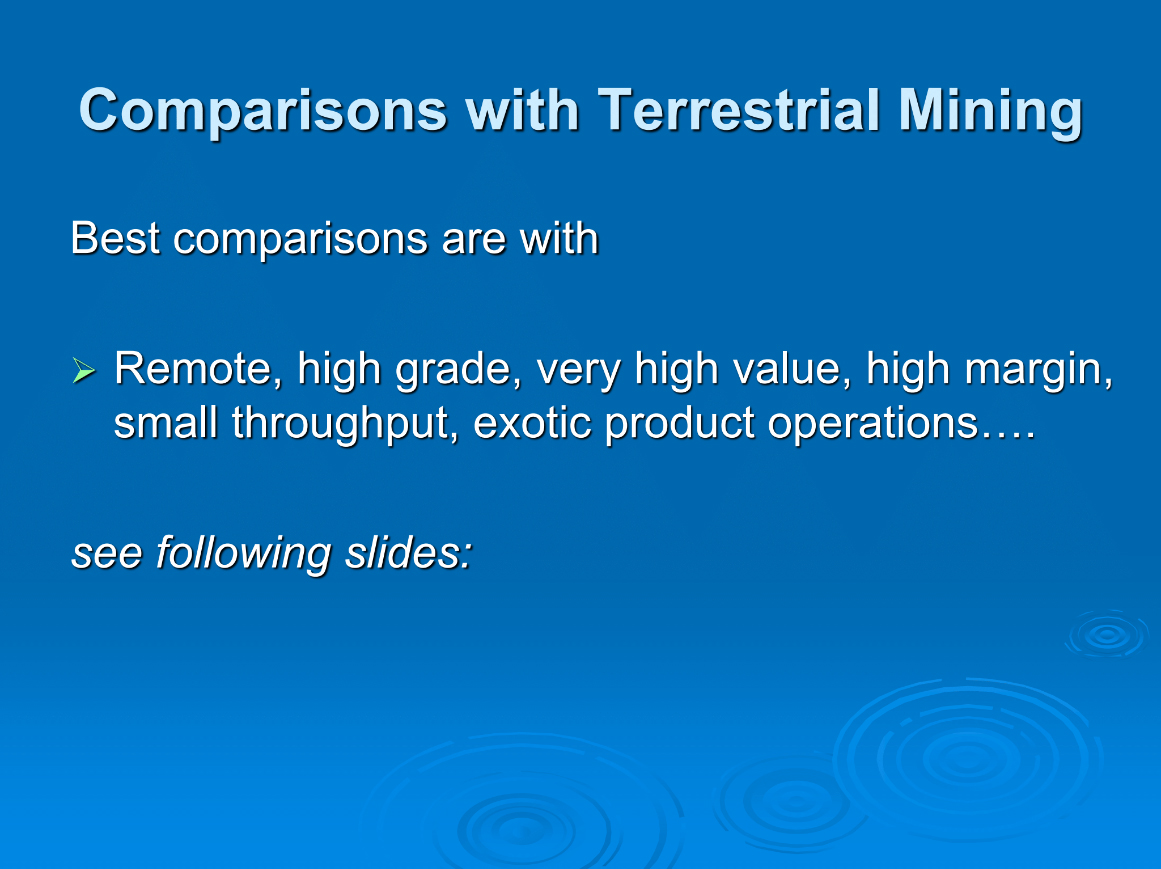
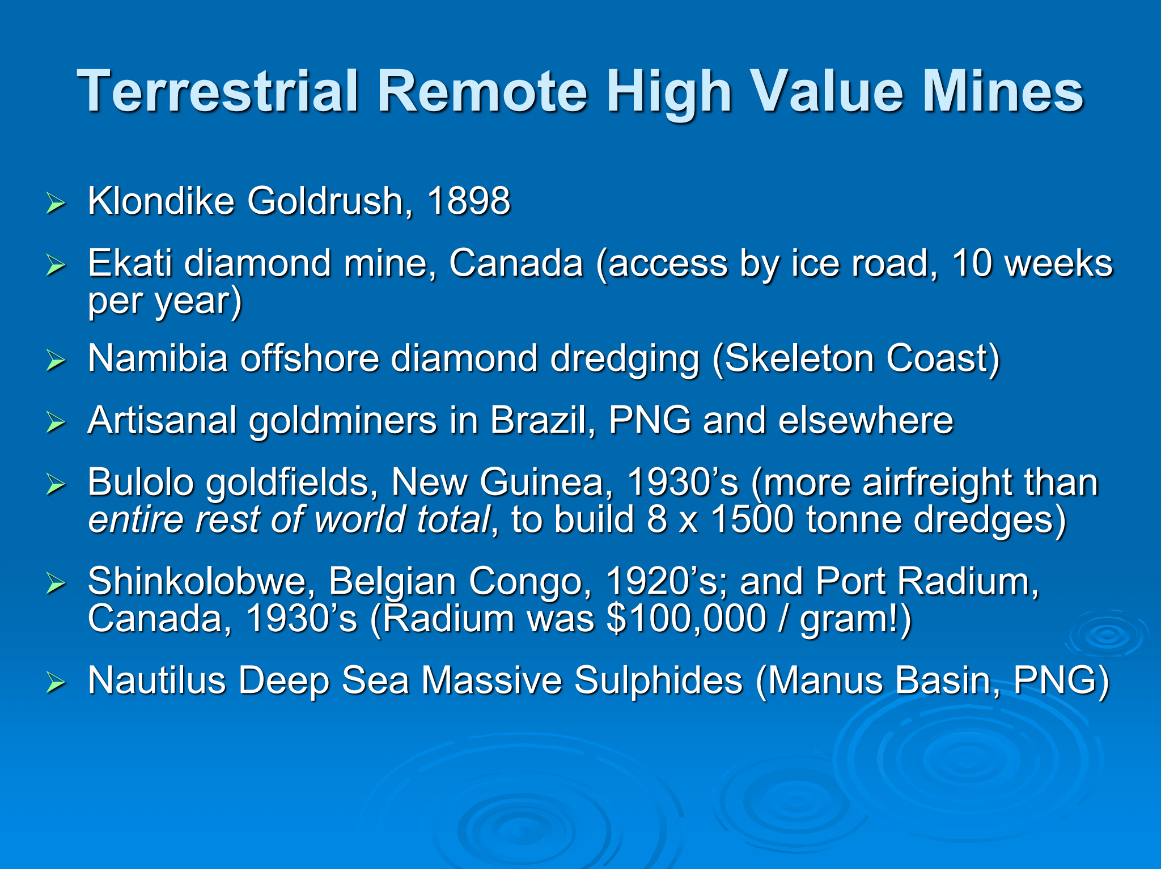
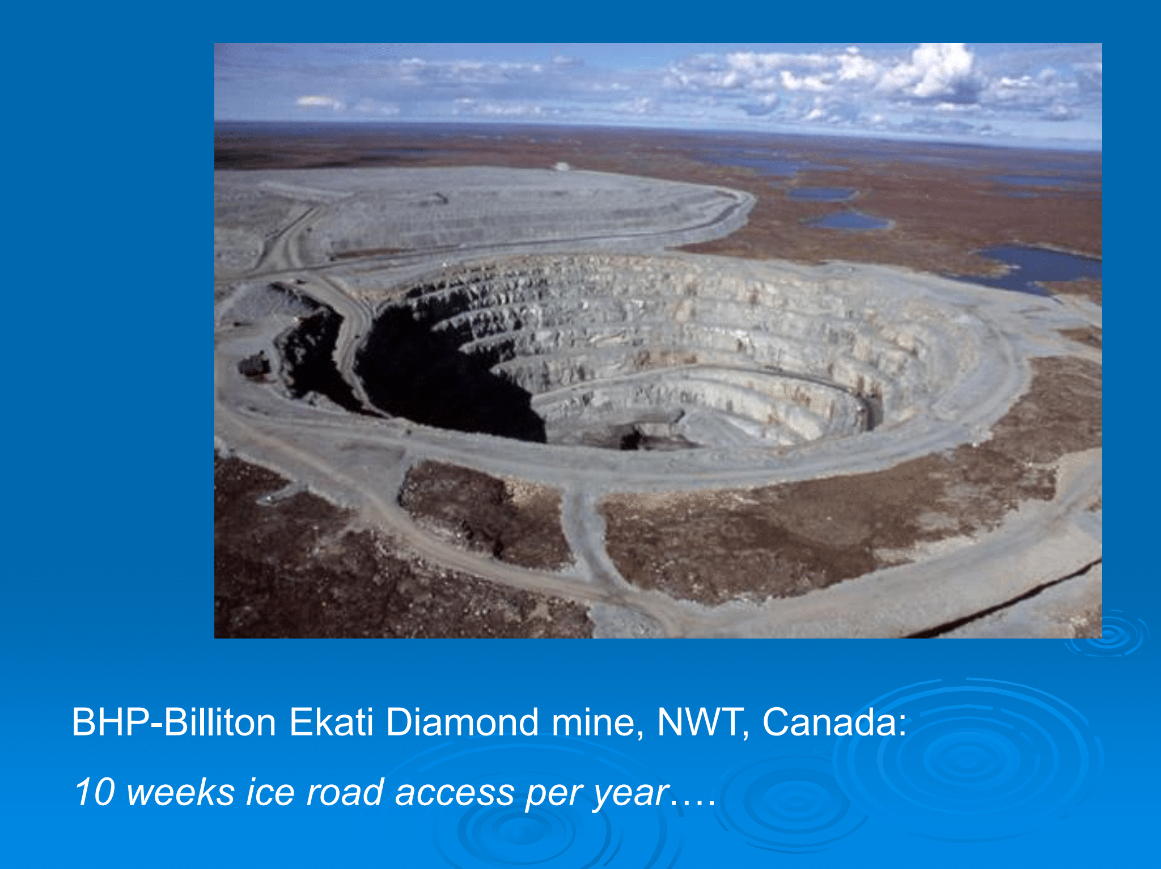
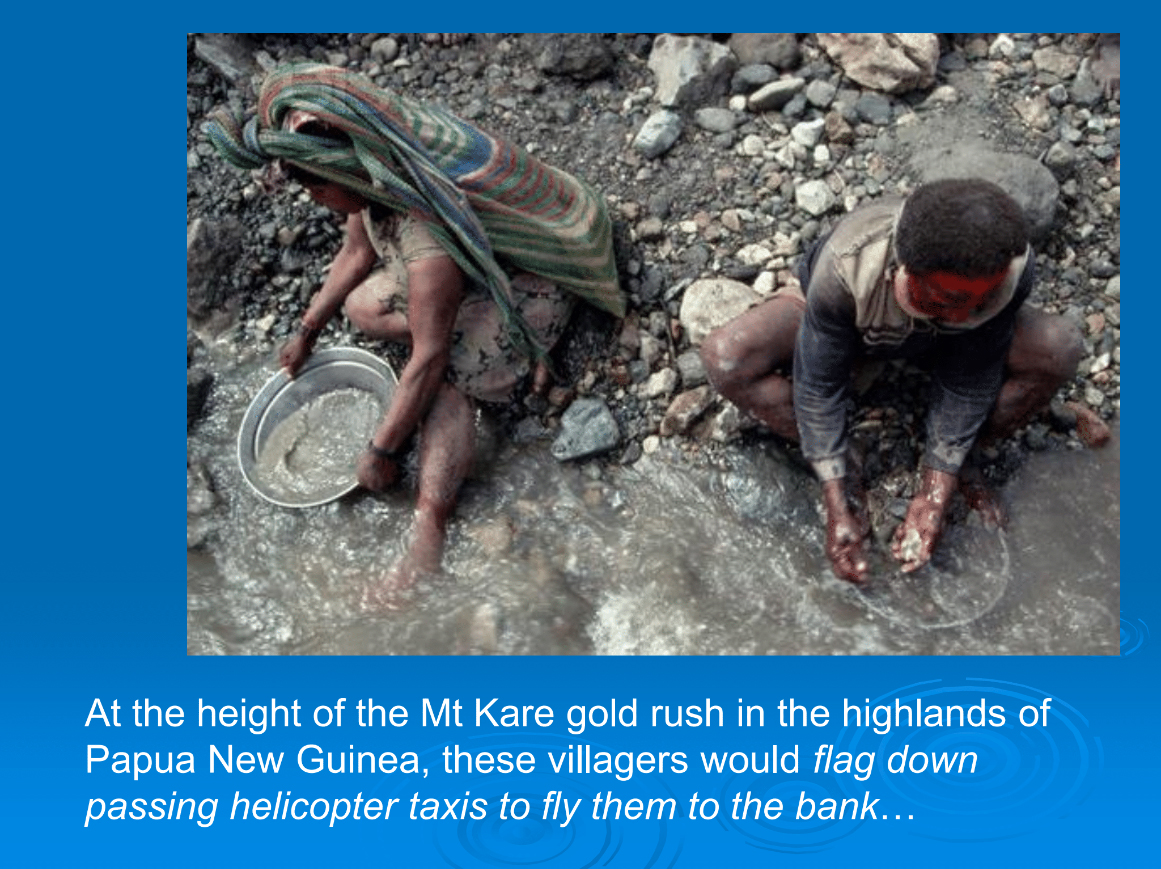
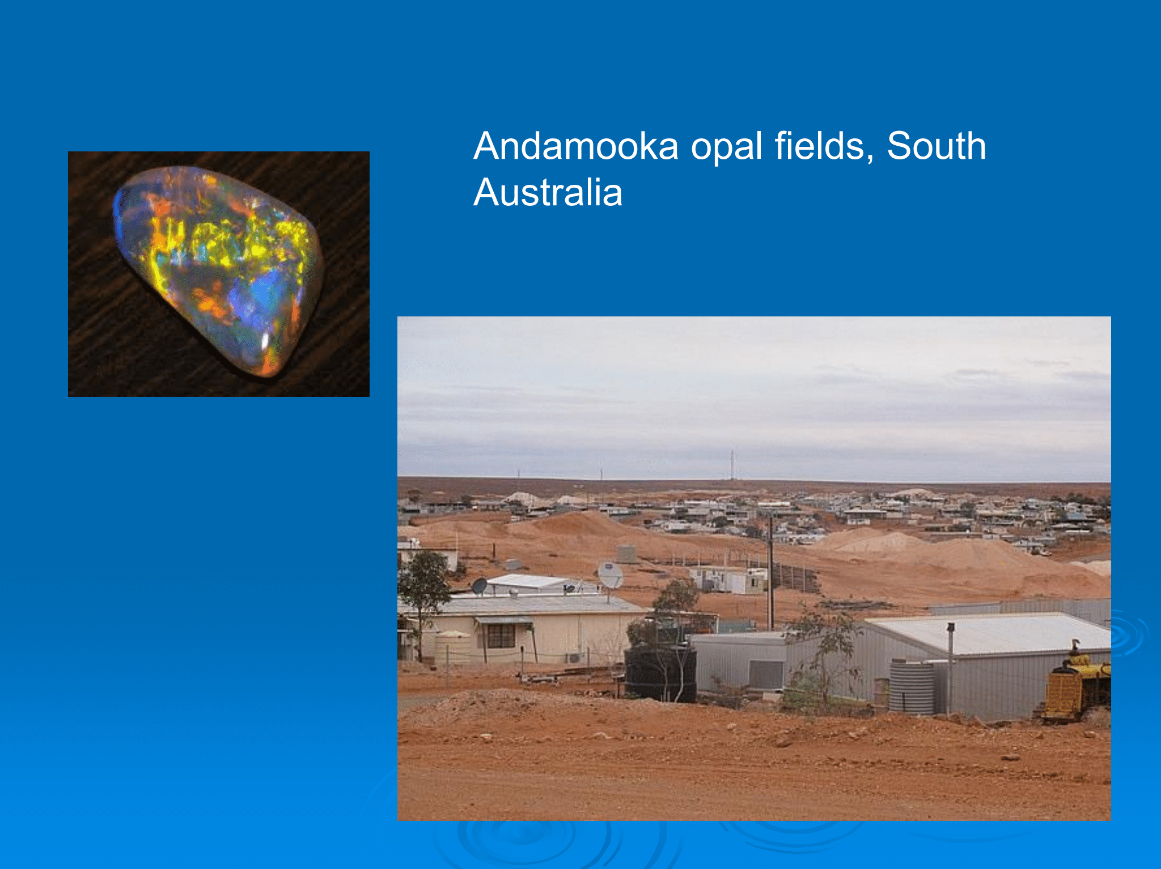
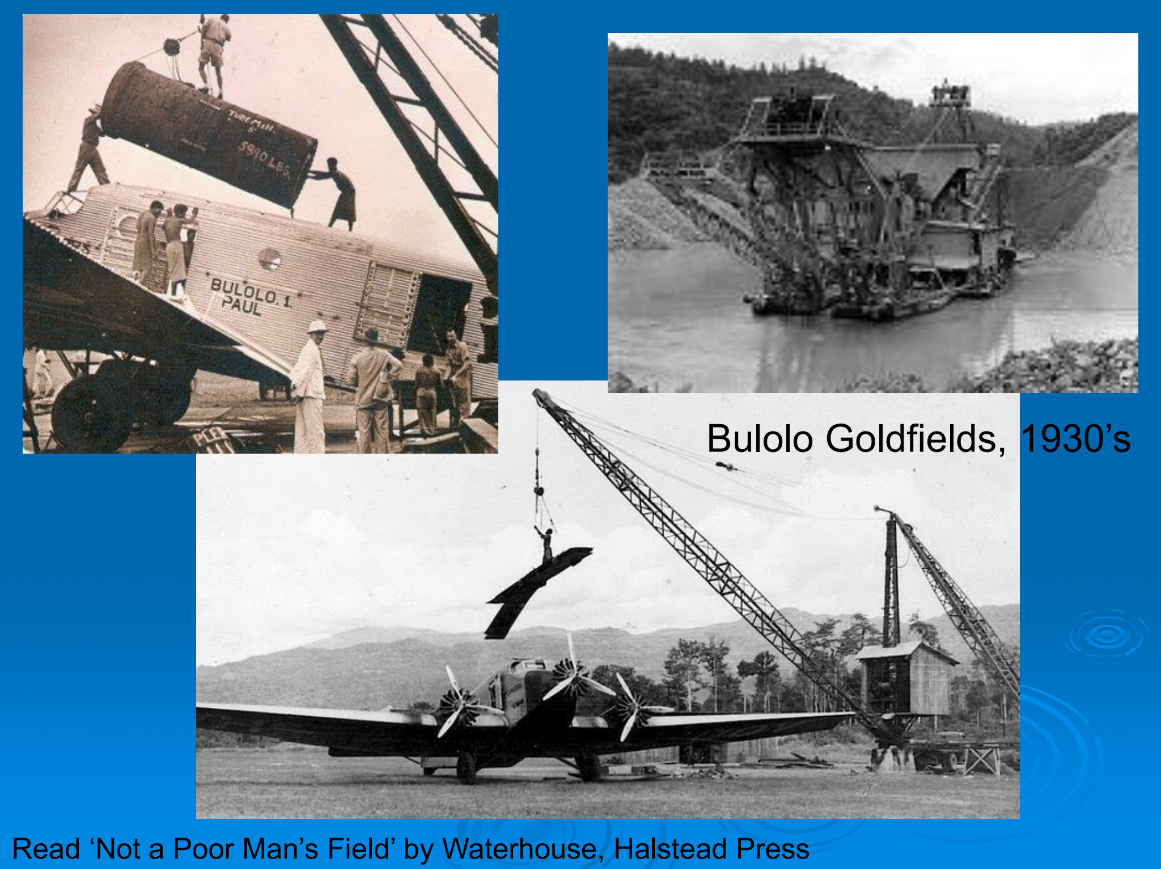
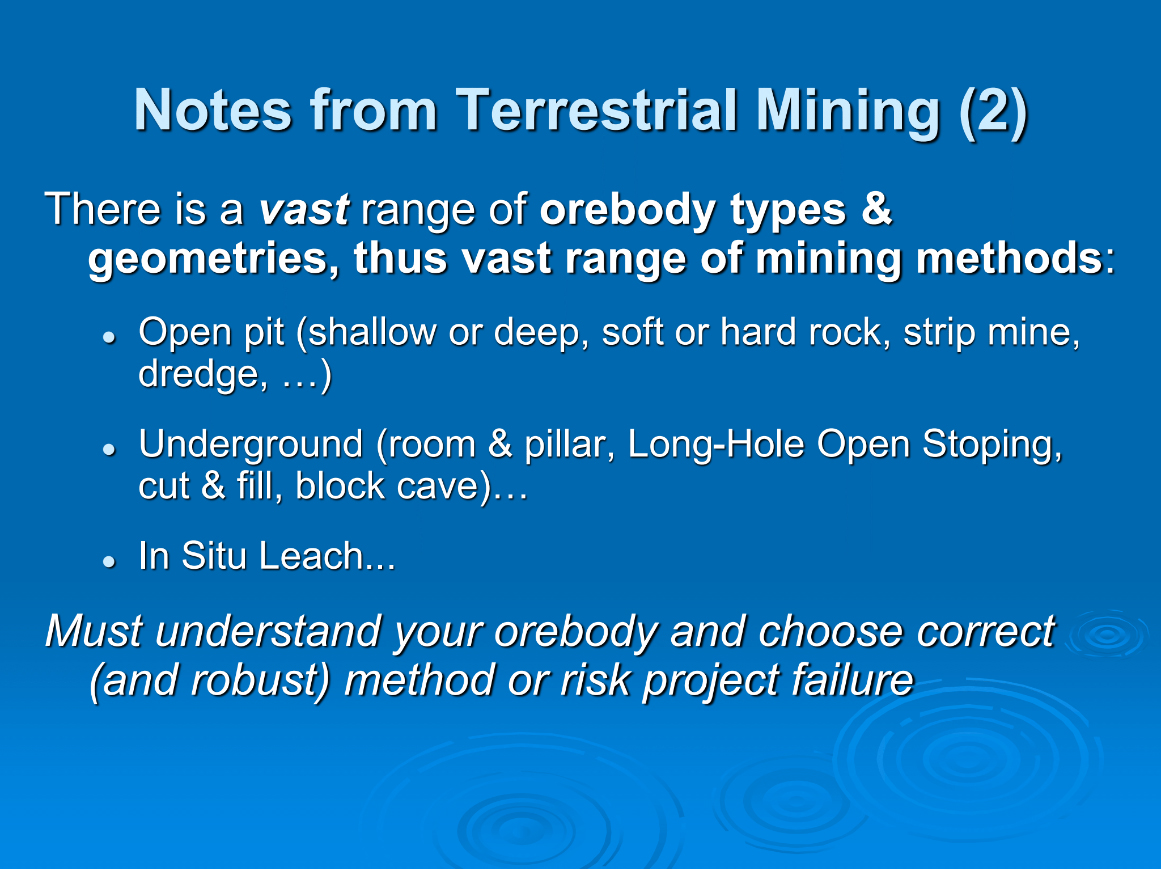
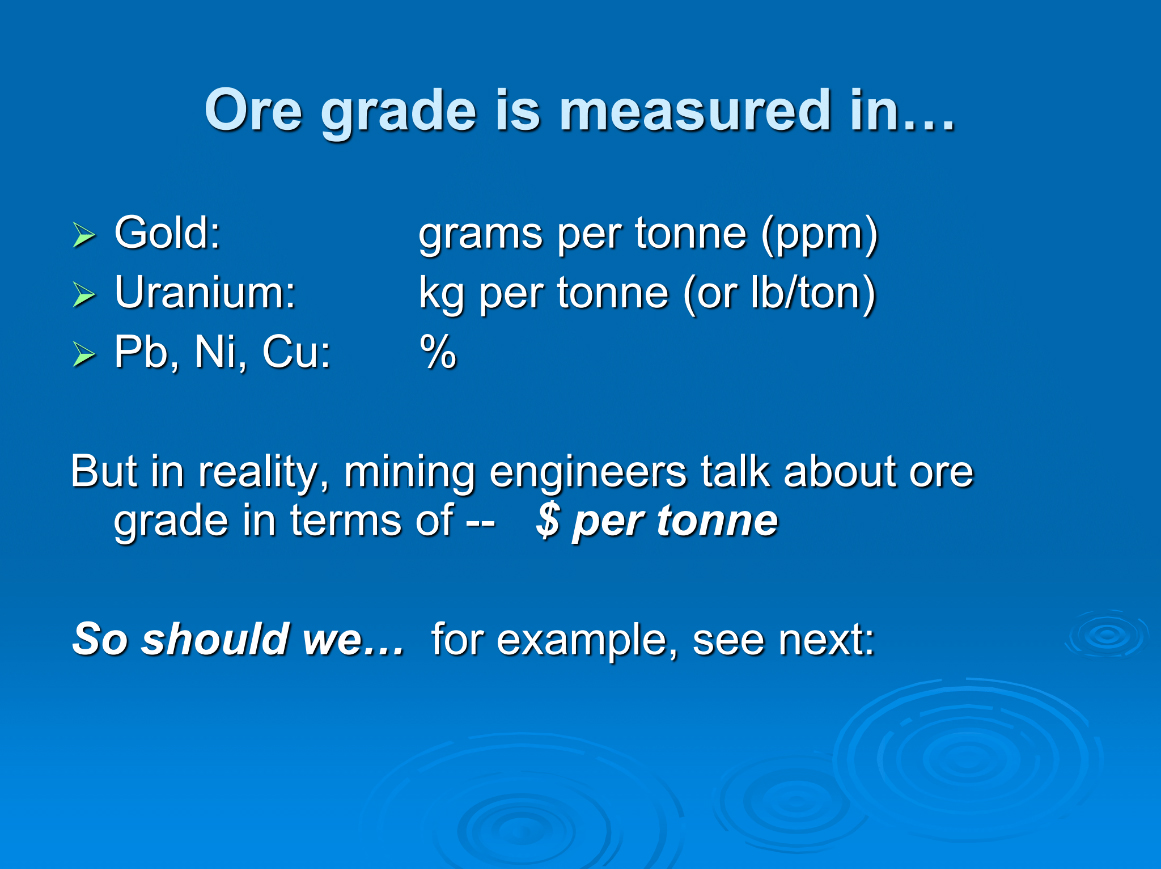
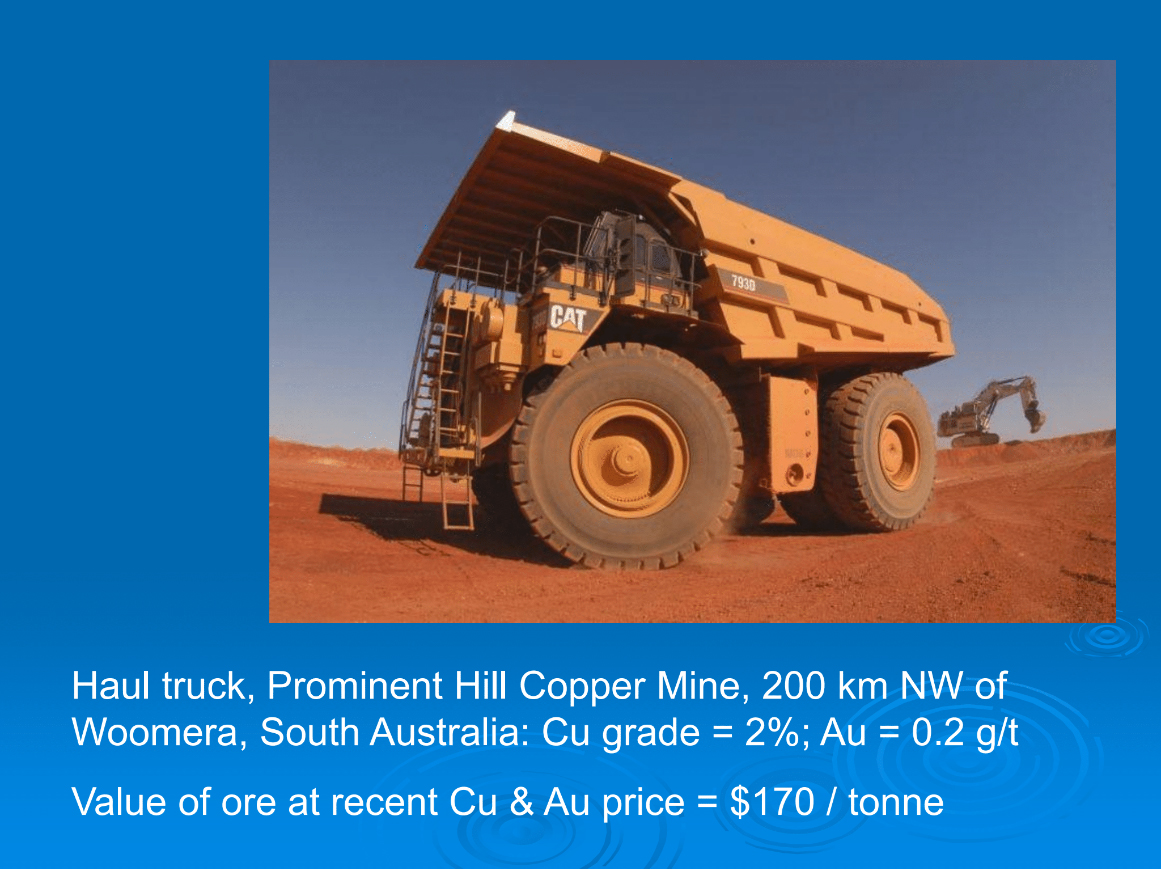
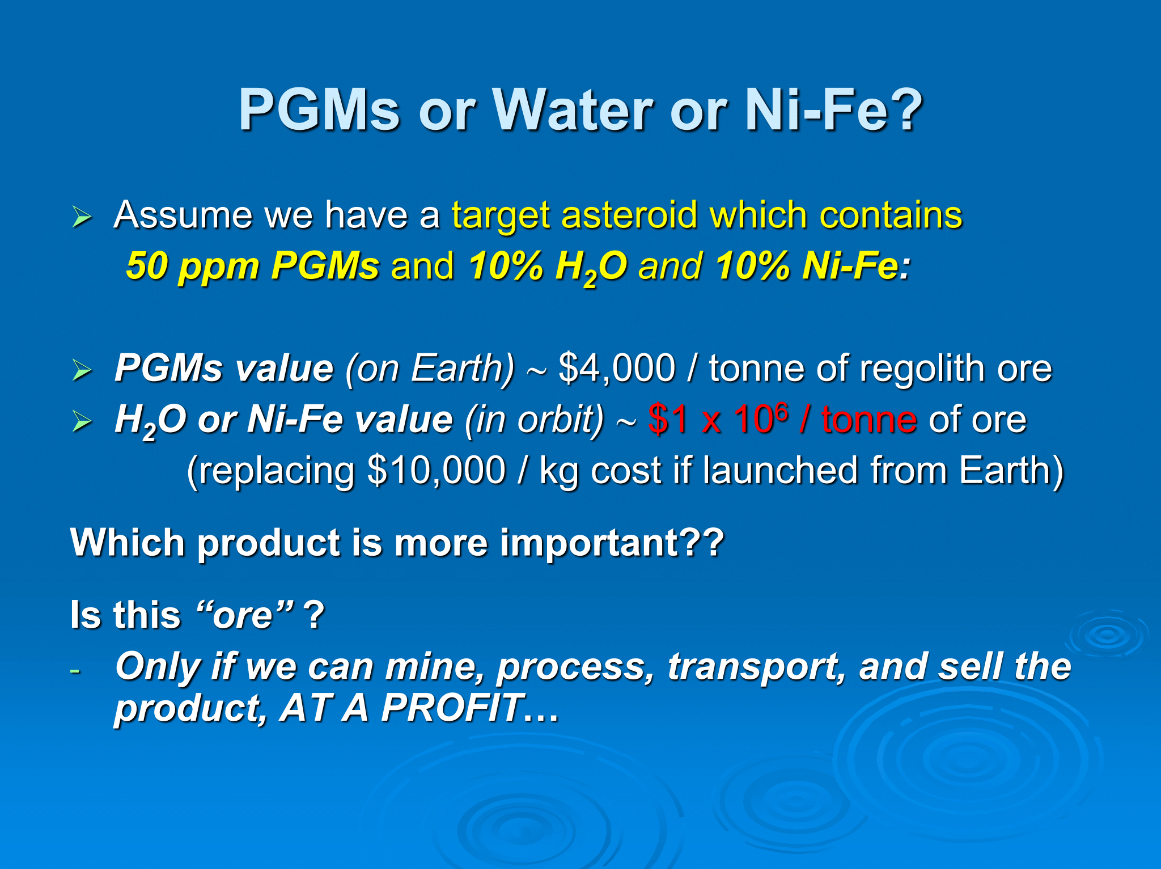
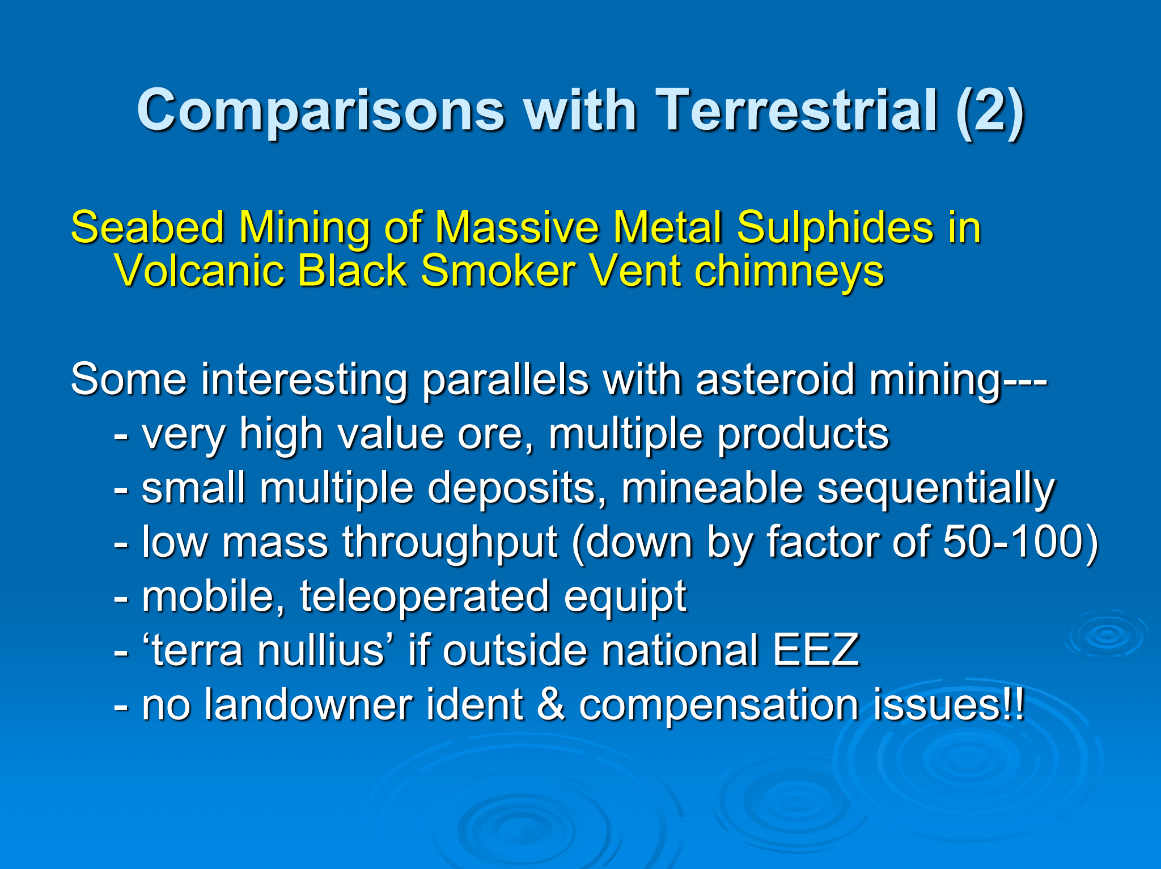
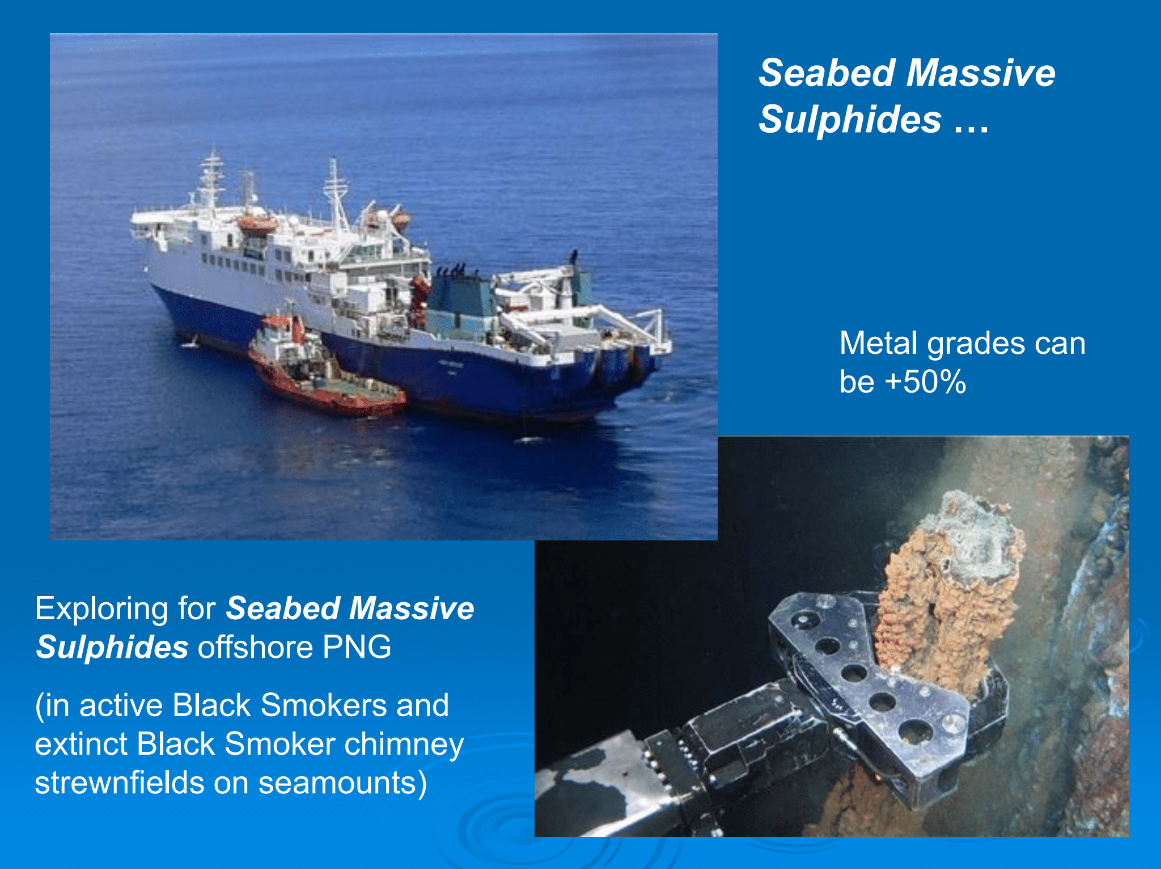
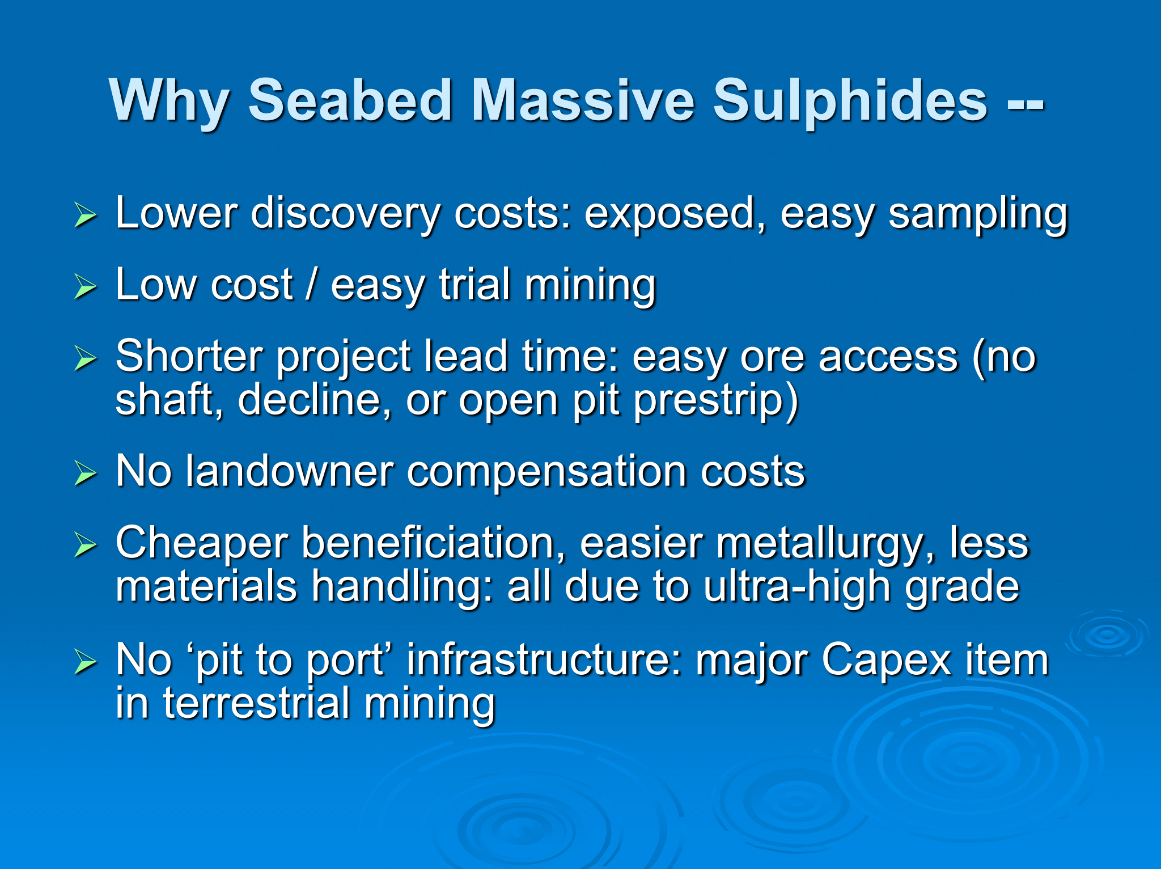
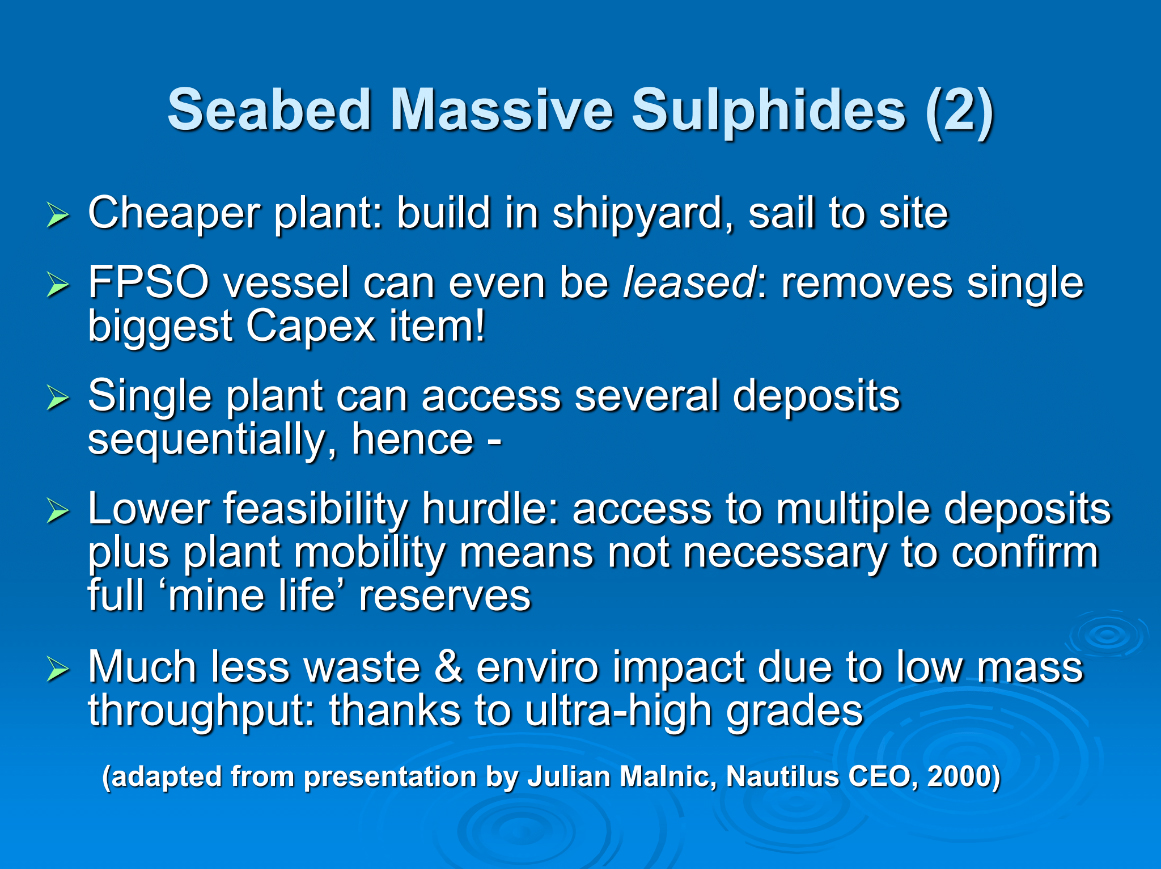
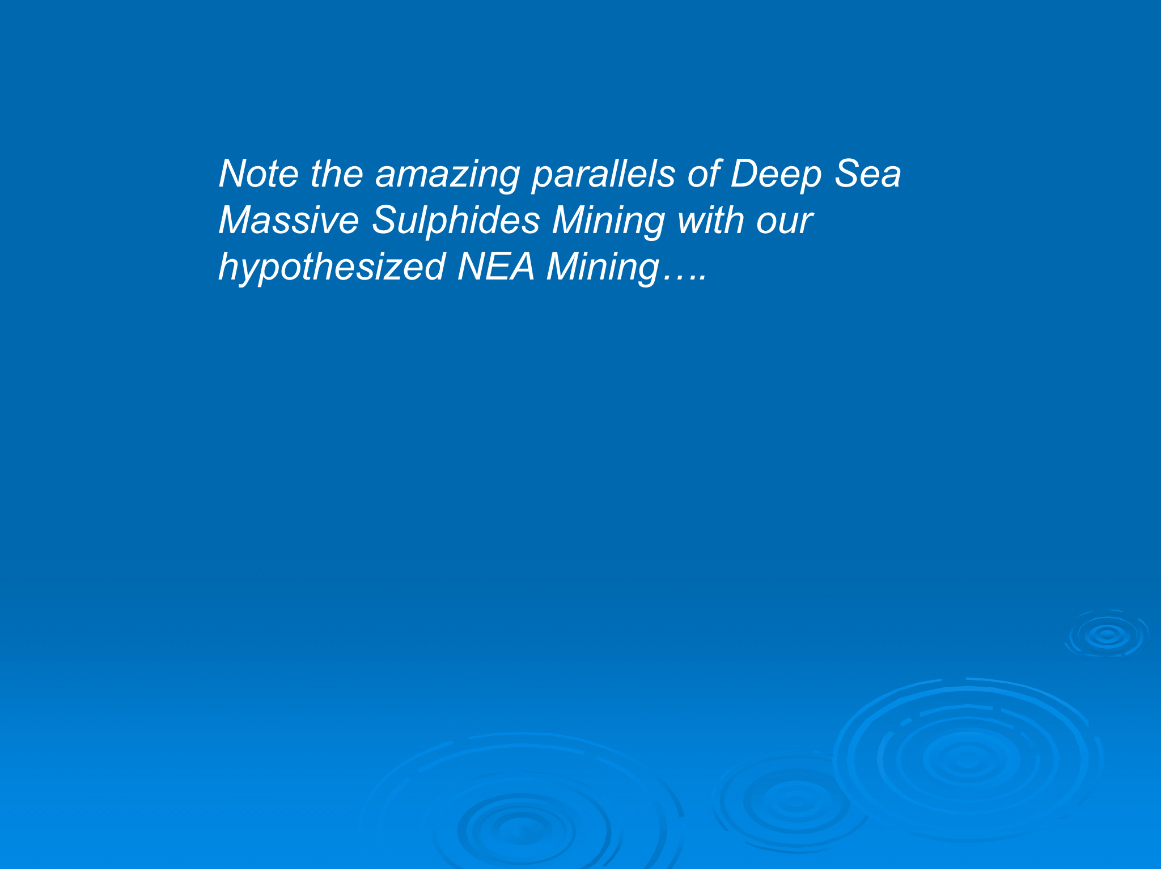
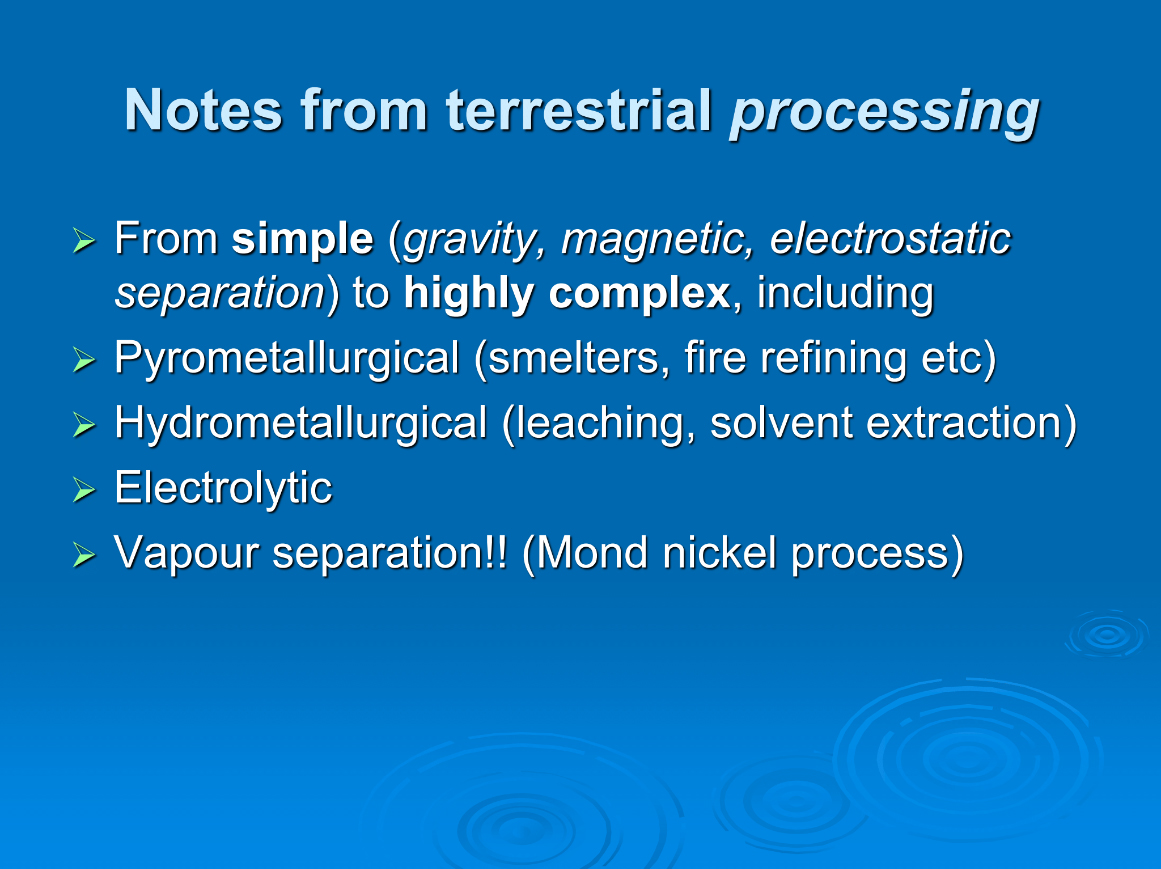
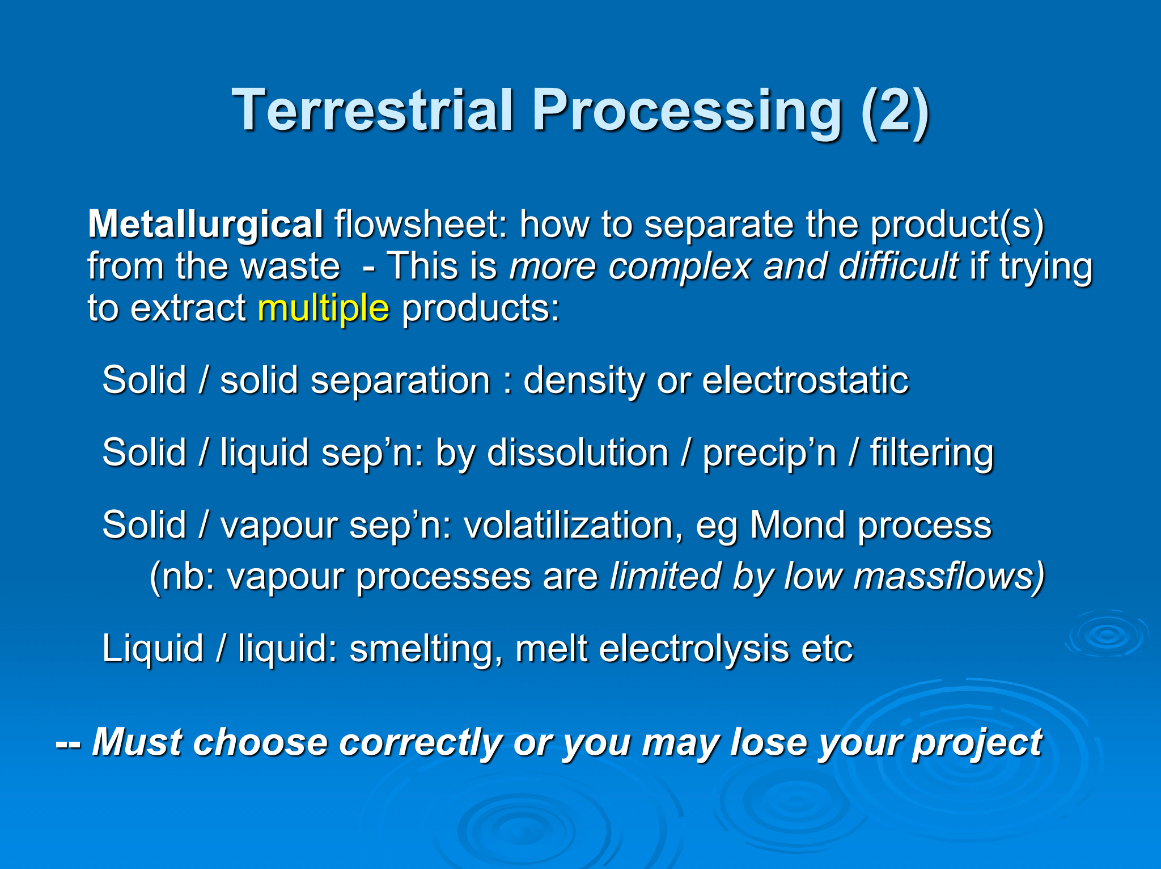
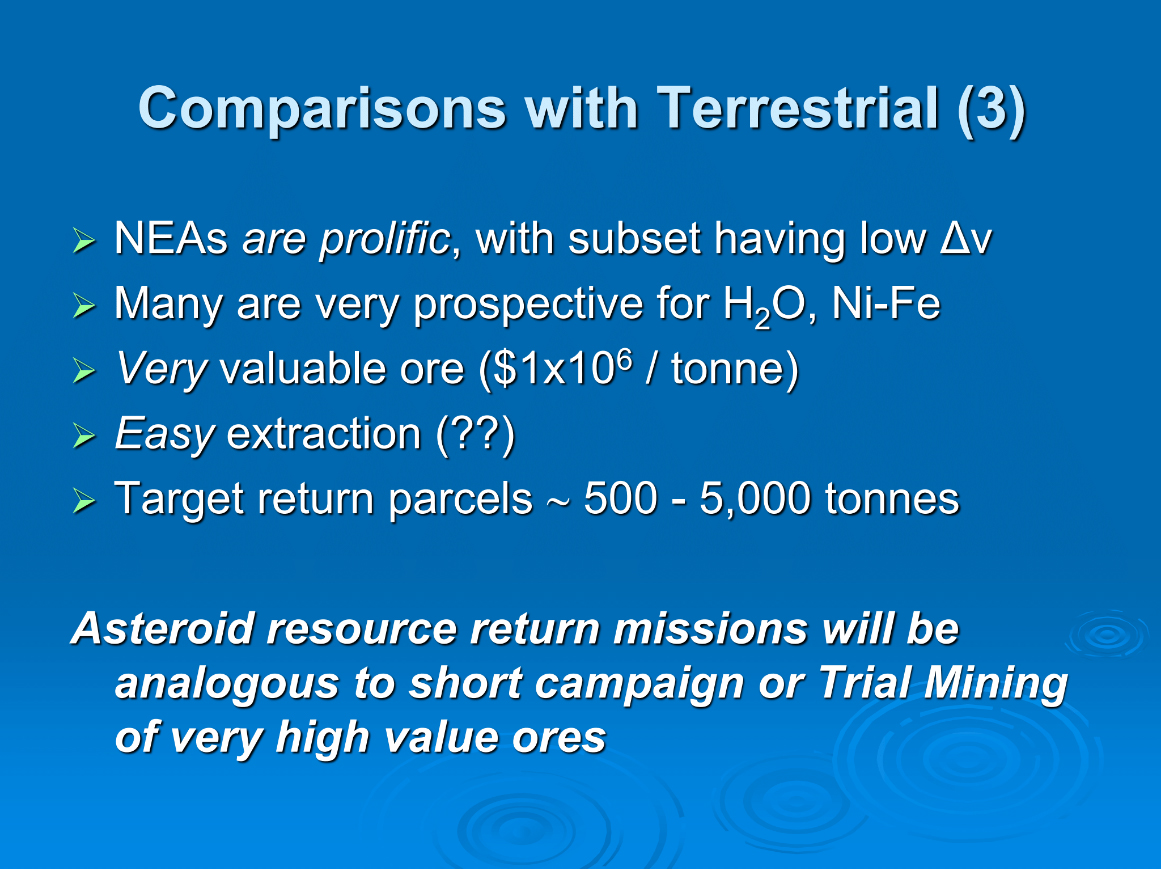
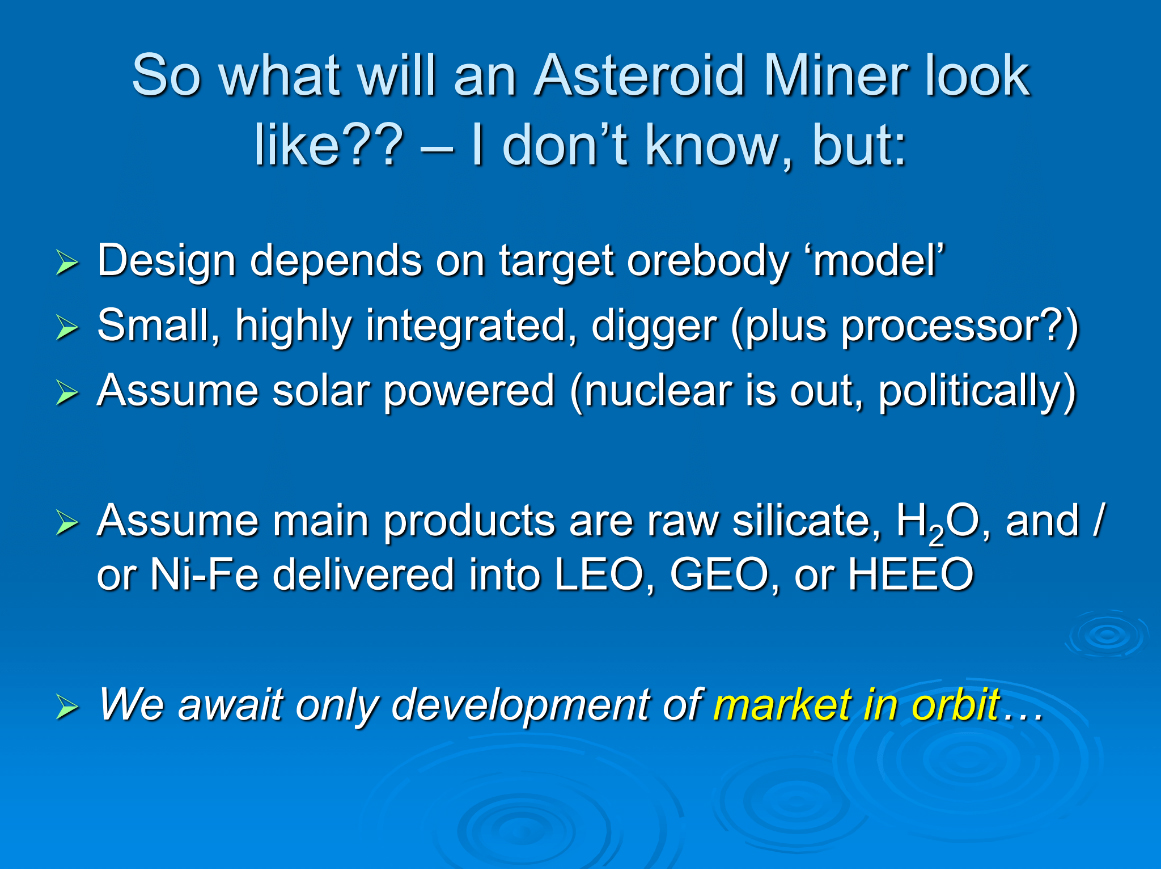
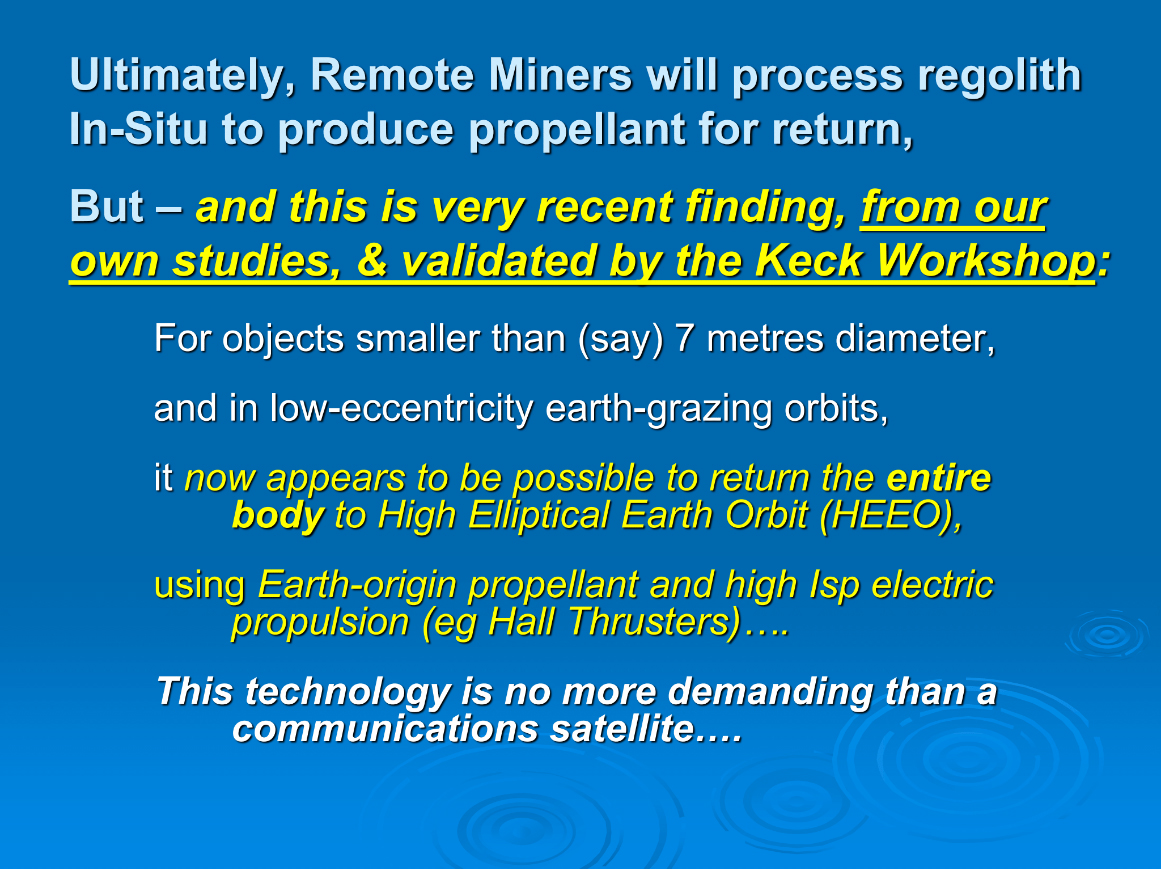
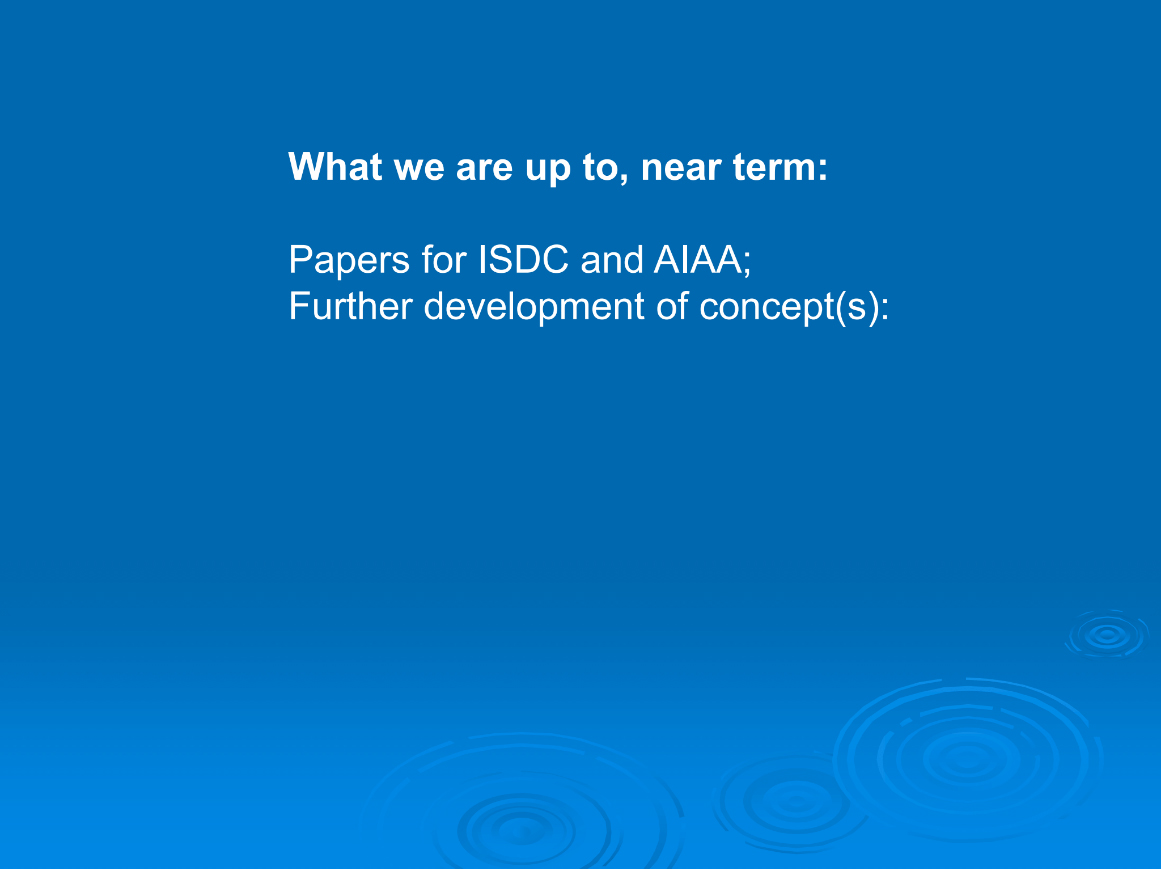
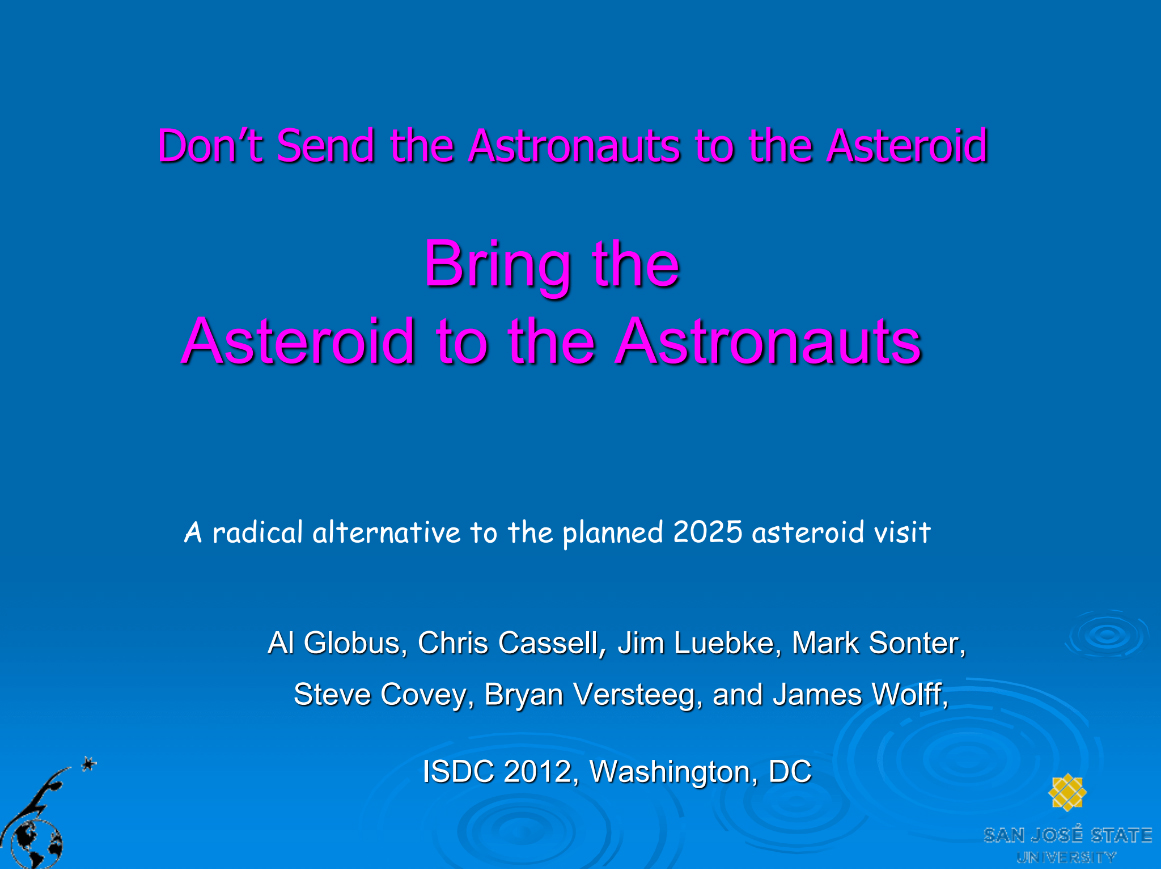
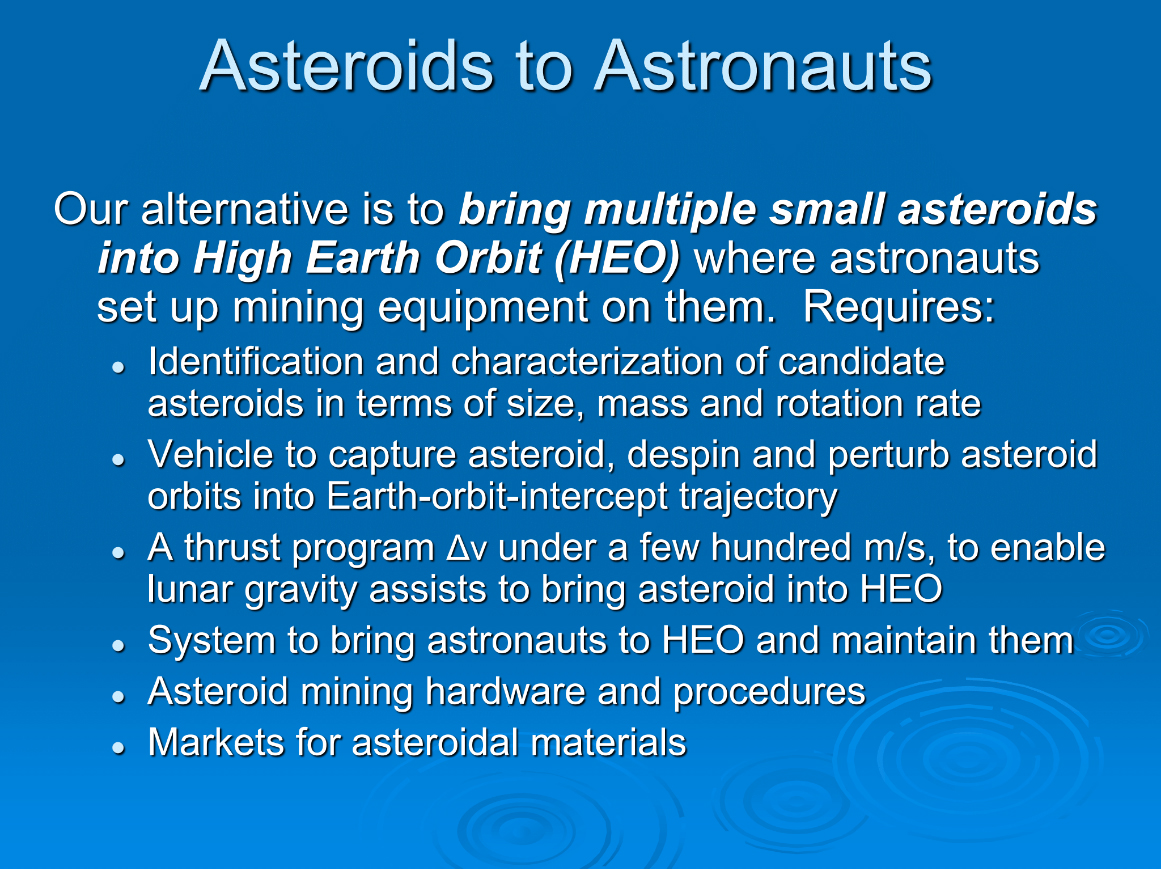
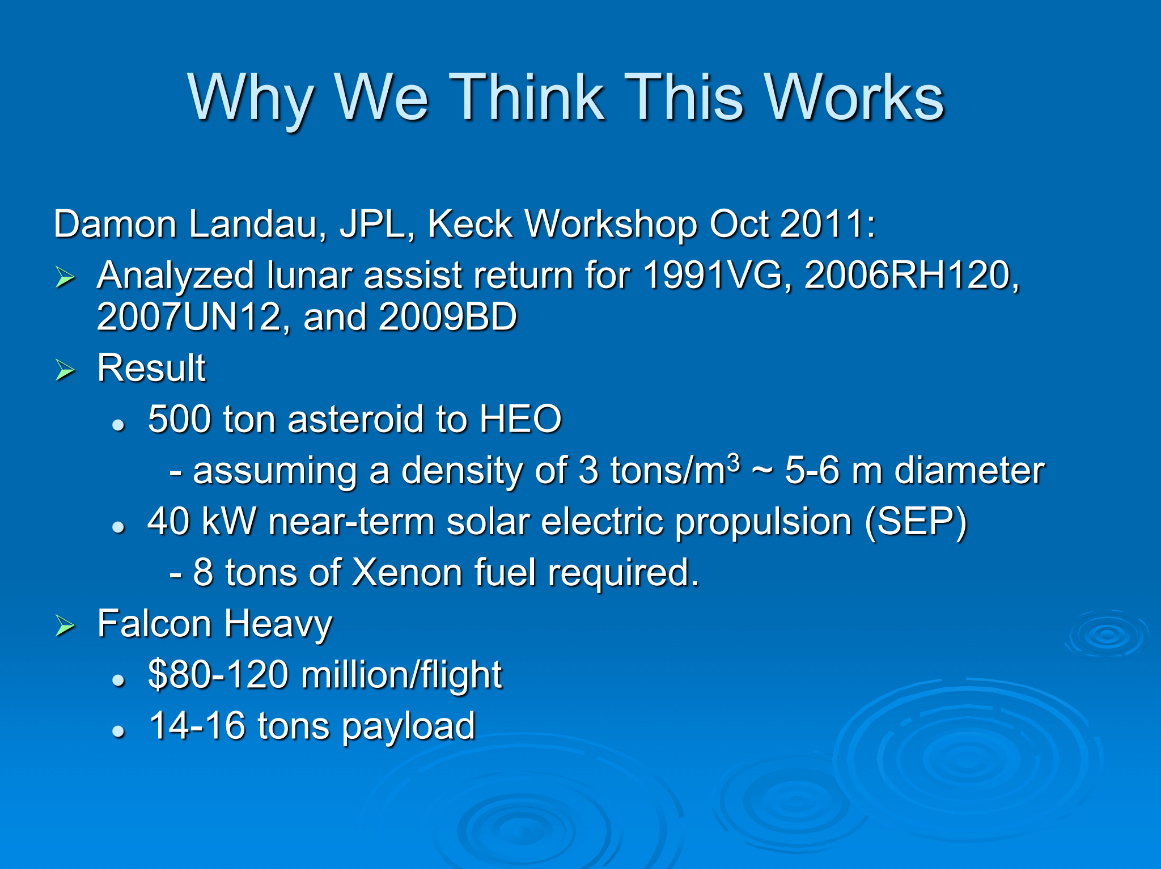
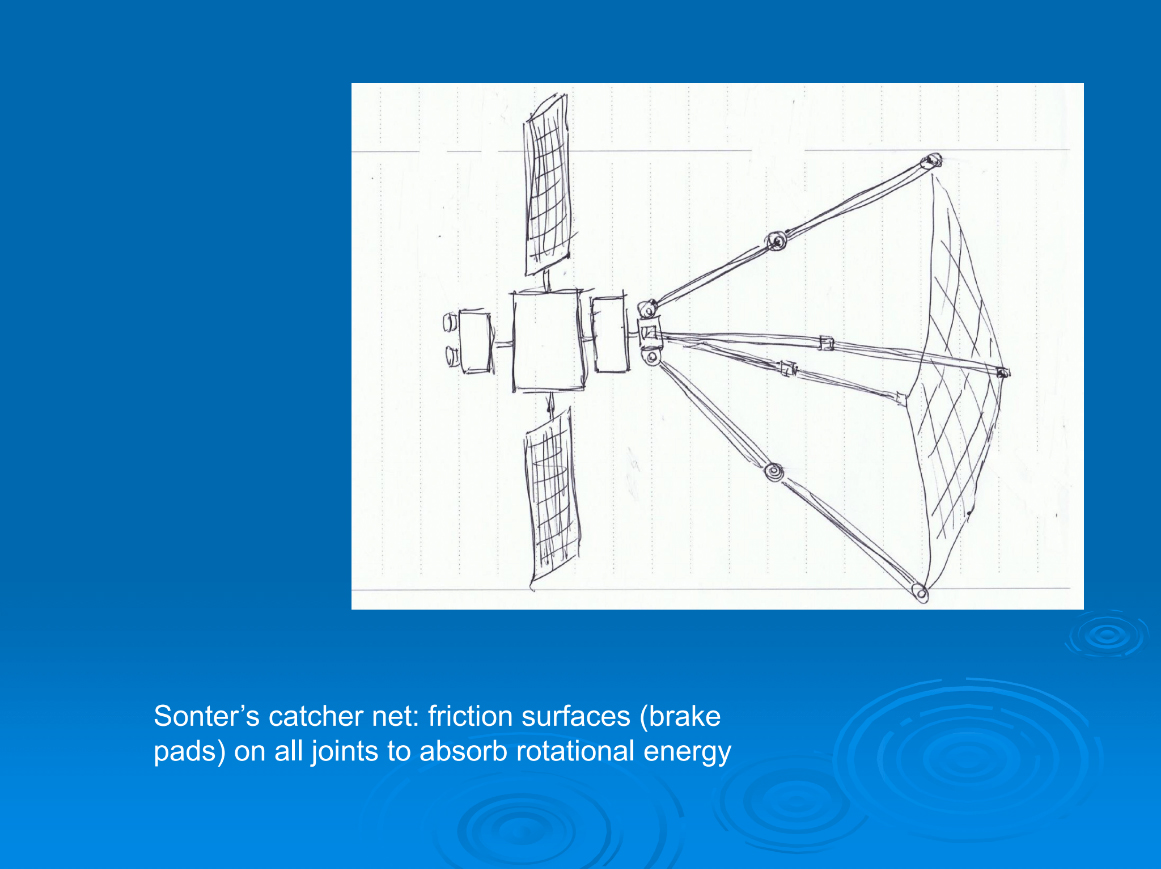
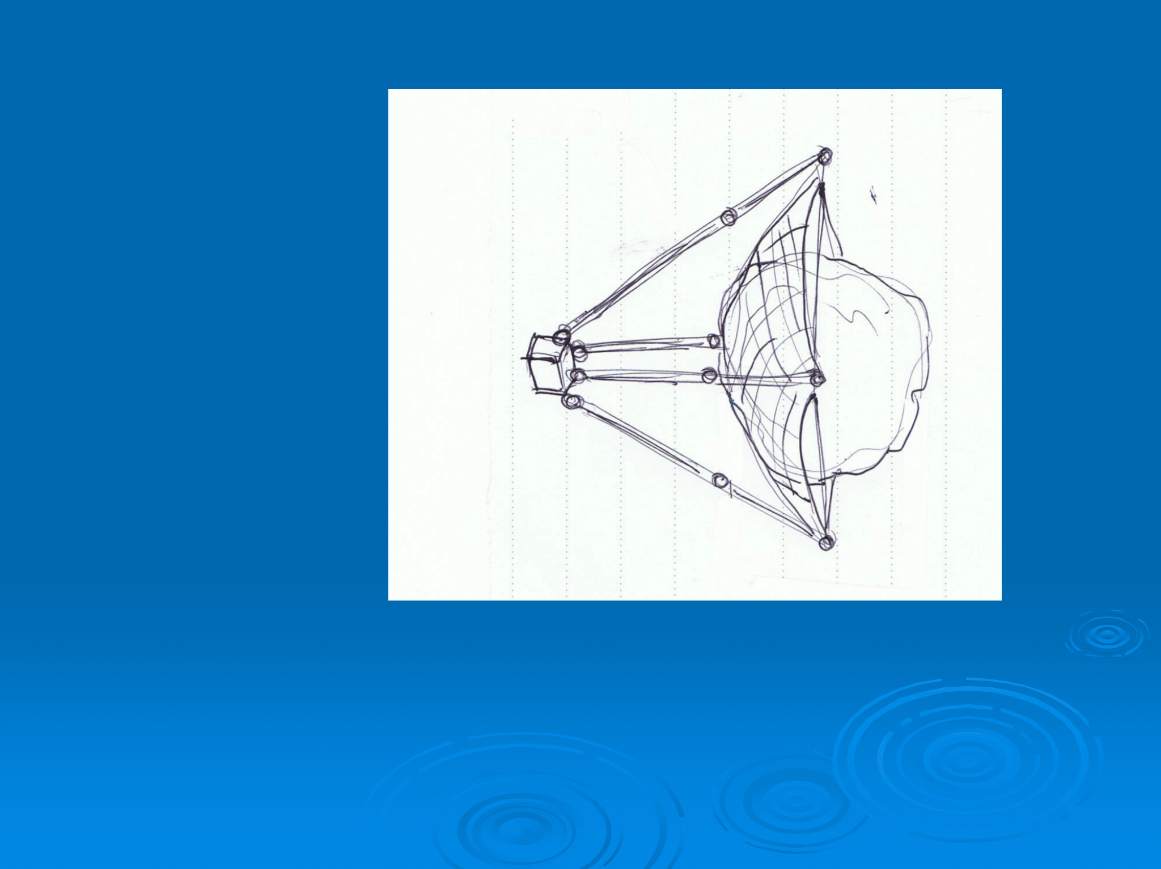
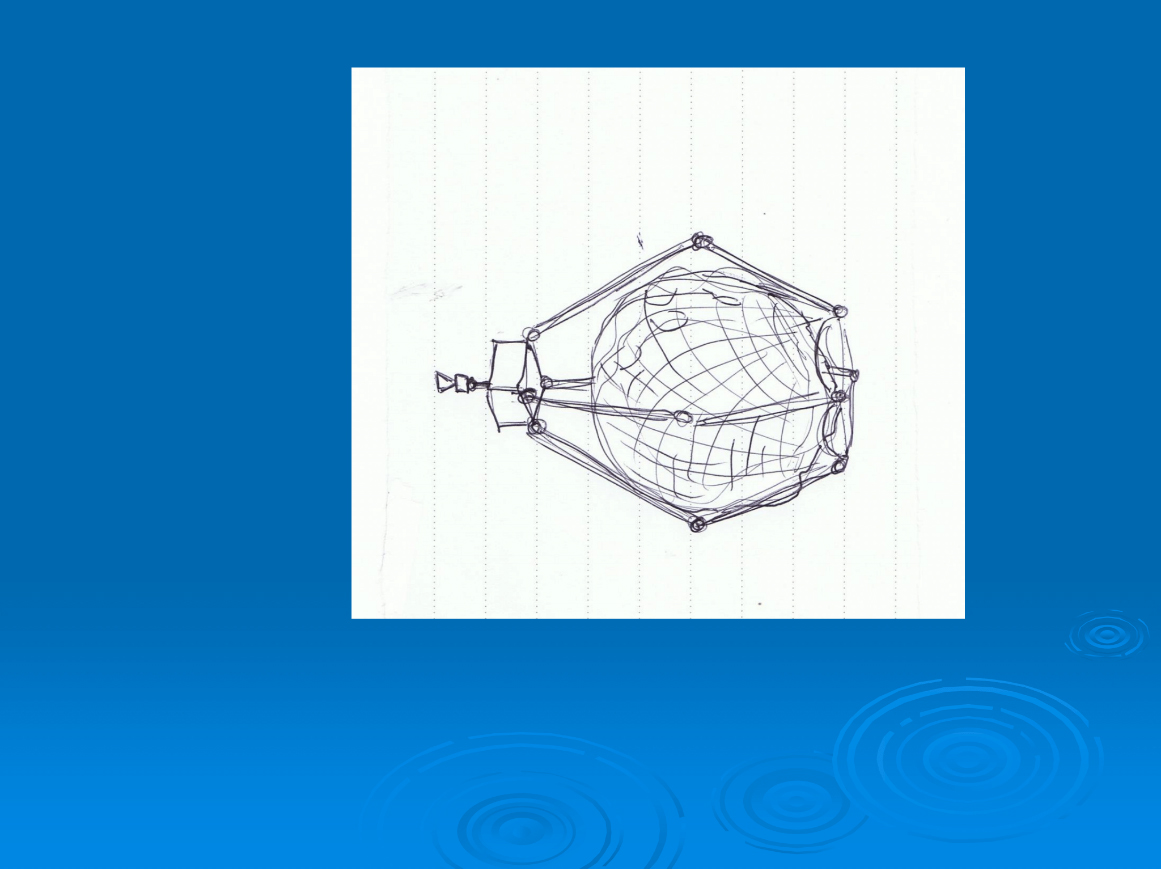
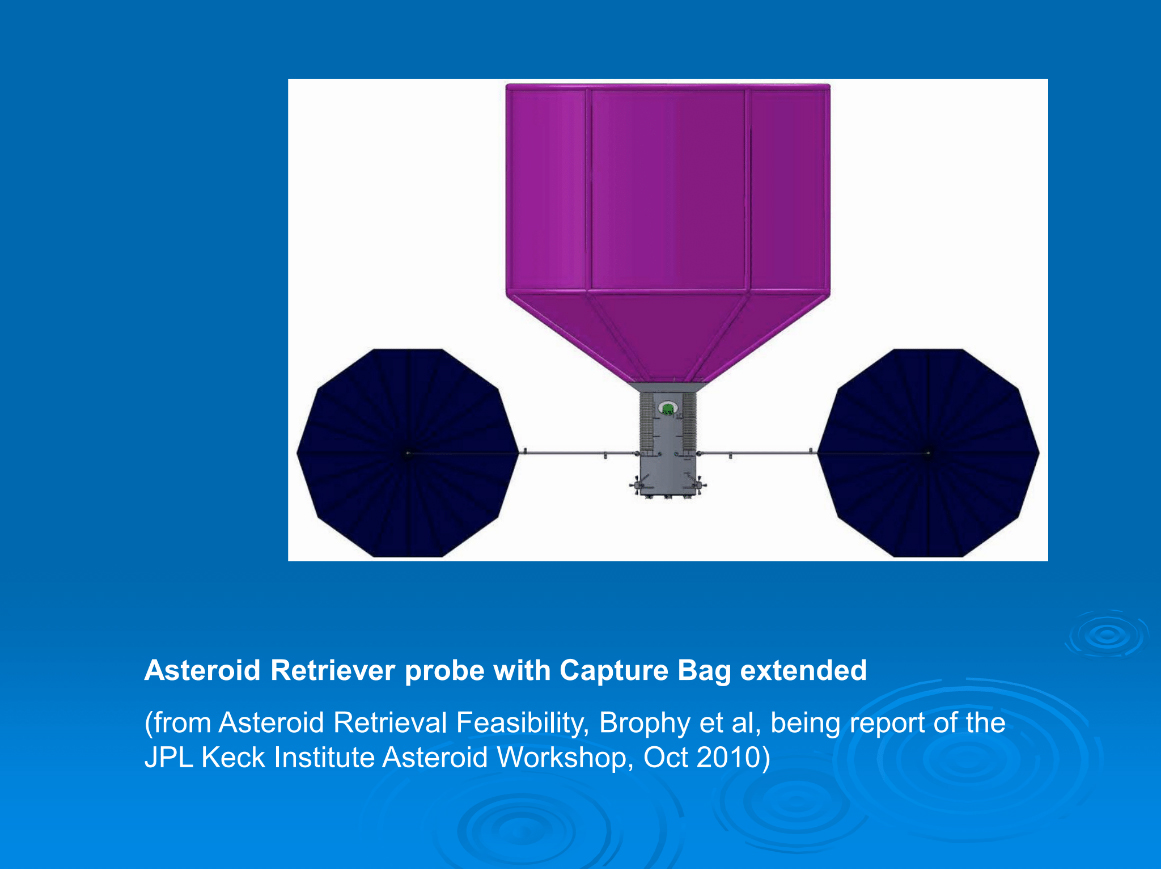
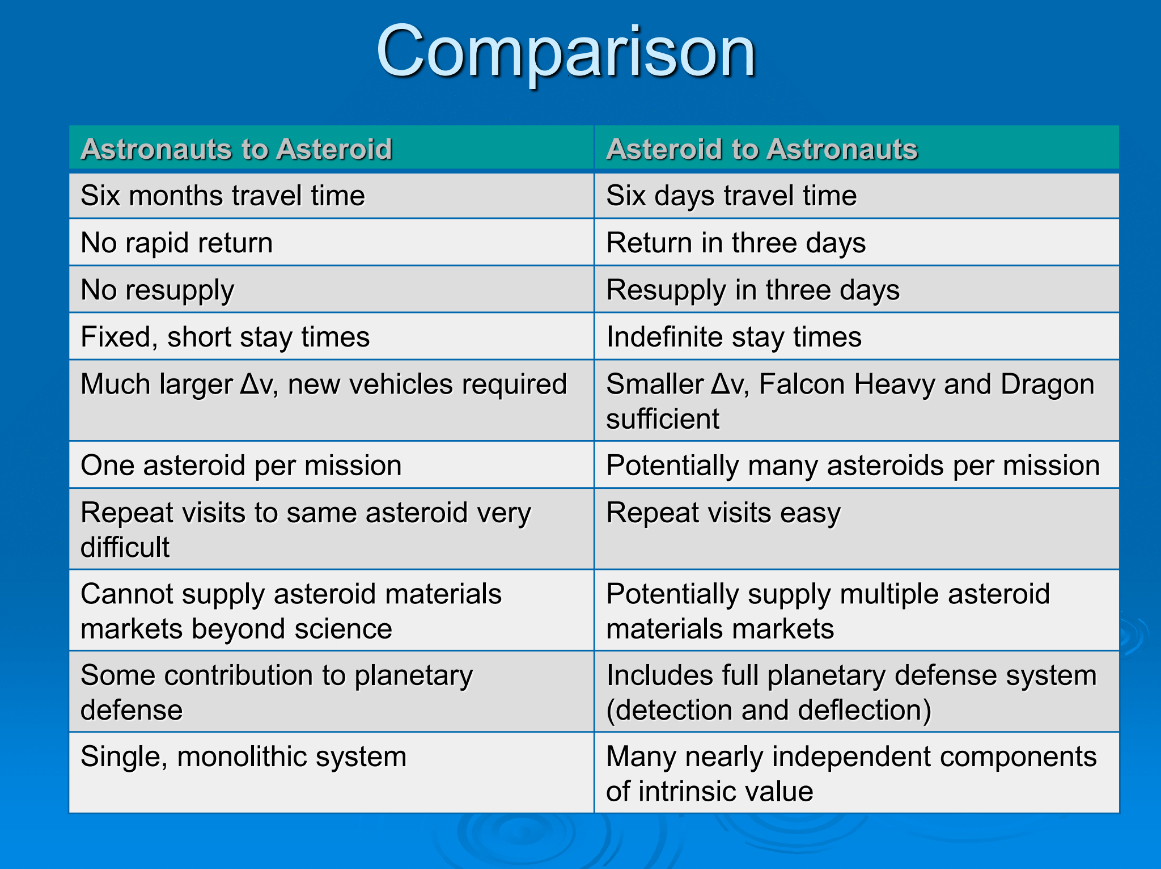
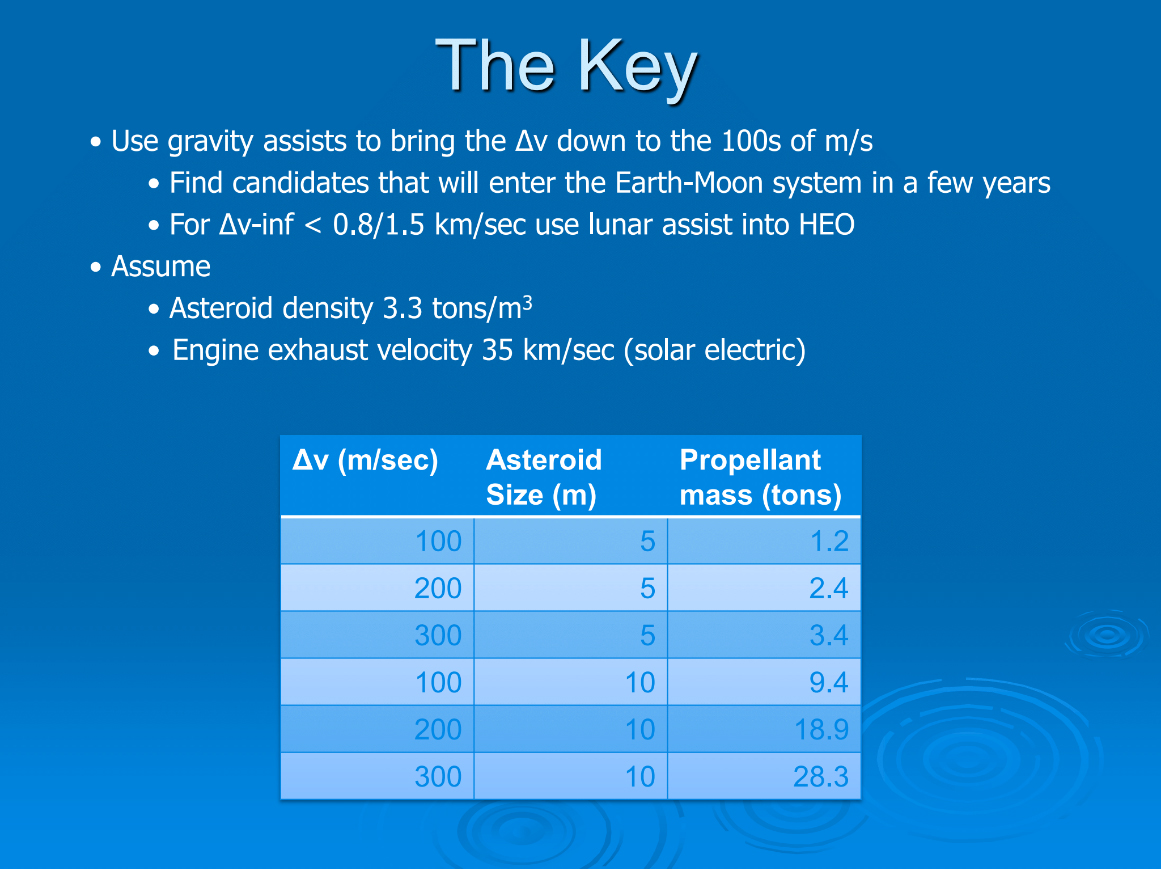
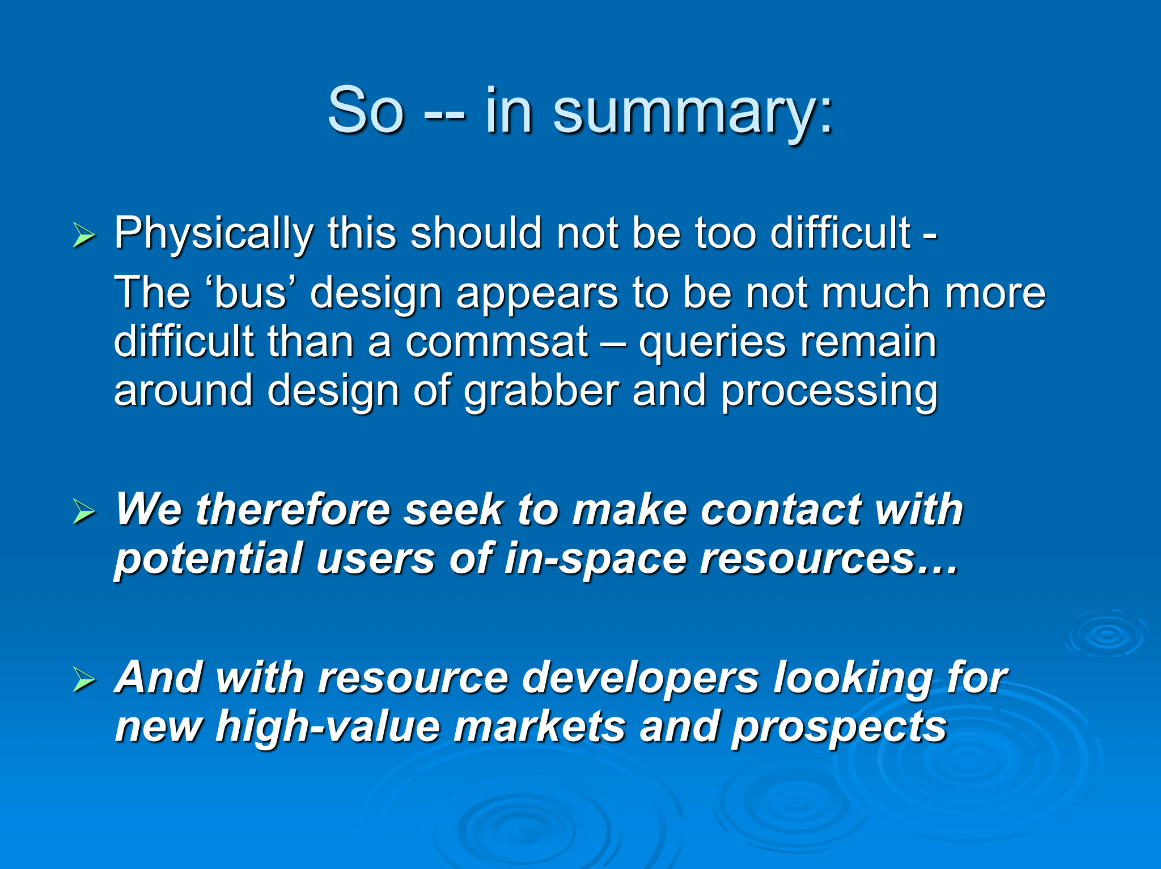
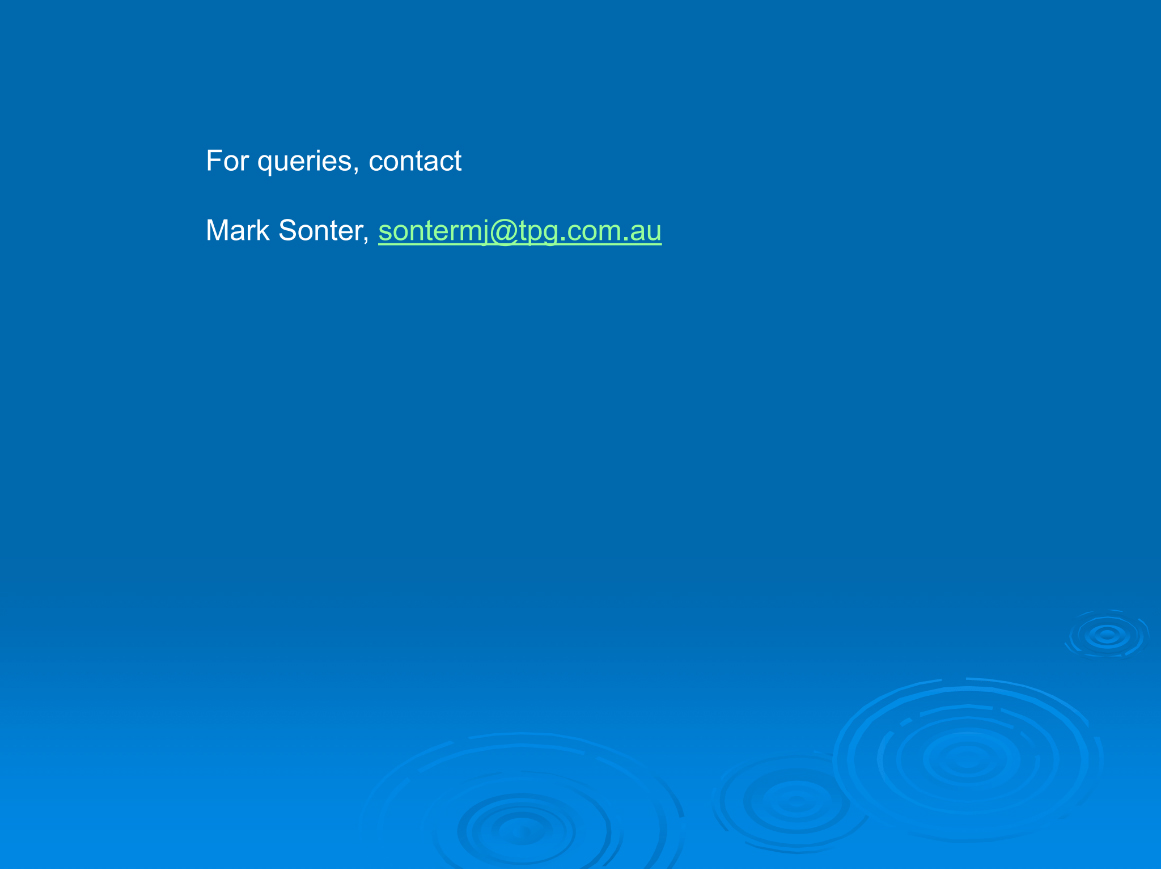
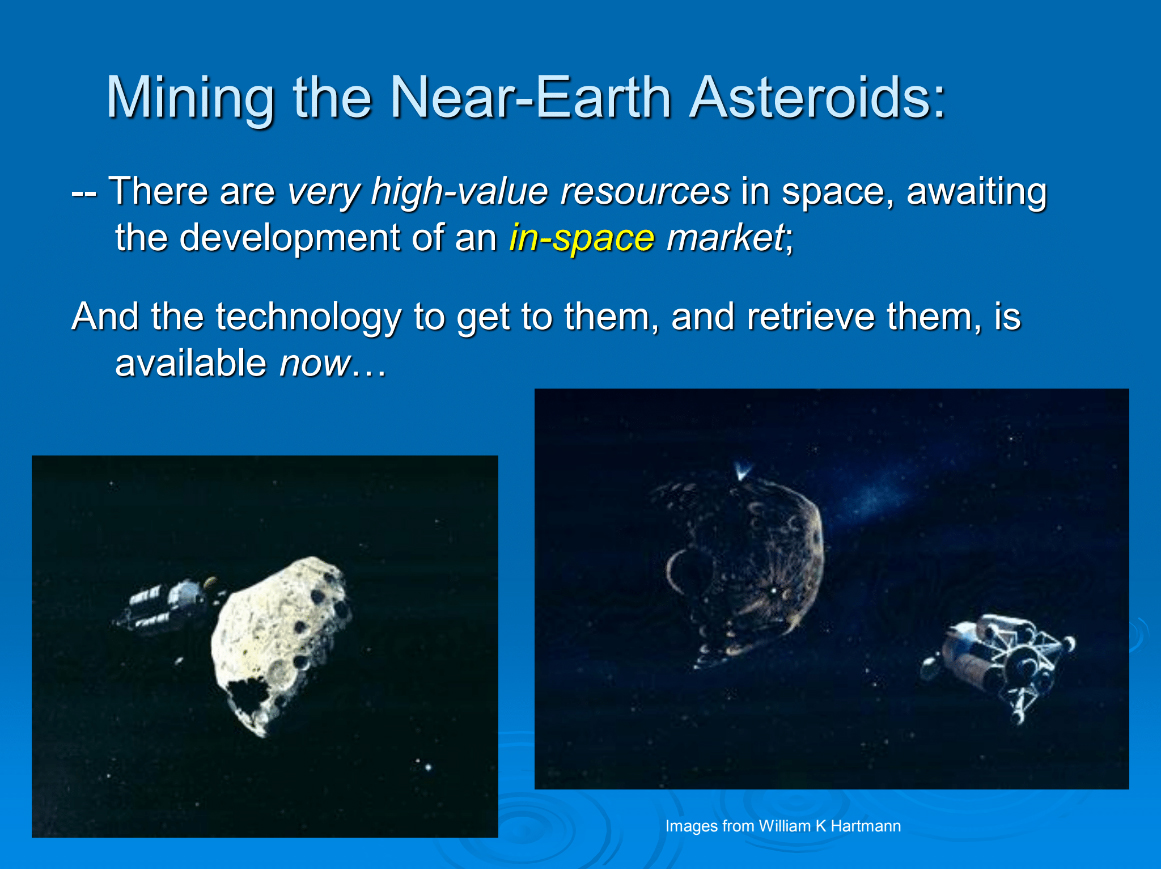