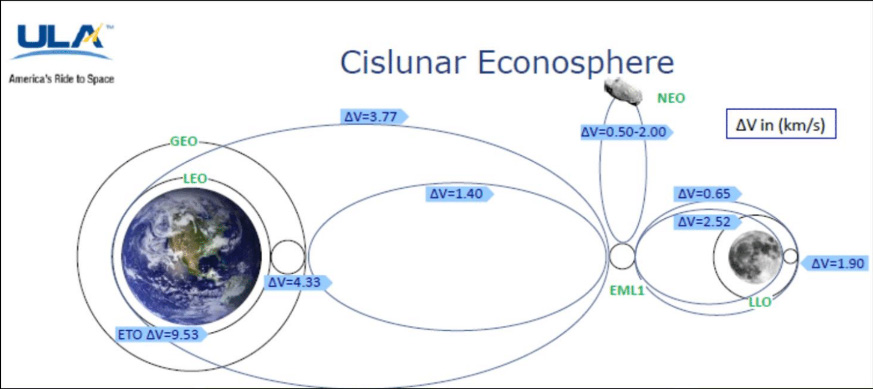
Moon vs NEAs: Resource Comparisons
Mark Sonter
Mining on the Moon, comparison with mining on asteroids
Mining engineering on the Moon was first seriously discussed at length in a ca. 50 page‘ Project Horizon’ Study undertaken by the US Army Corps of Engineers, in 1959. Focus was on excavation of regolith for use as radiation shielding and insulation cover over prefabricated habitat modules.
Concepts for automated mining equipment for use on the Moon (front end loaders, trucks, etc) powered by electrics / fuel cells have been revisited many times (see eg various papers by Brad Blair). The automation aspects have been detailed most fully by Greg Baiden of Penguin Engineering (this is the fellow who developed automated Load-Haul-Dump operation and truck haulage in the underground Inco Nickel operations at Sudbury in the 1980s).
Potential resources:
Low-grade but ubiquitous resources in regolith:
- (i) ilmenite, anorthite, other feldspars, KREEP in mare regolith. There were proposals in the late 1970s / early 1980s to reduce ilmenite using imported and recycled hydrogen to extract oxygen for rocket fuel (‘Lunox Corp’: Davis, and others). The reaction chemistry is straightforward, but the assumed front-end magnetic concentration as proposed by Agosto probably would not have worked as expected (pers comm, J S Lewis)
- Various overly optimistic comments are found in the literature regarding the rare earths and thorium contents of KREEP: these need to be treated with great caution: the grades are very, very low, and the resource while potentially useful for local on-Moon consumption, eg thorium for nuclear power, could never compete as an export commodity delivered to Earth. Rare Earths(and Th) are far, far, far easier to access on Earth than mining, concentrating, and returning from Moon. “First thing to learn about Rare Earths is that they are not rare. ”And of course, thorium is ubiquitous on Earth in heavy mineral sands.
- (ii) solar wind volatiles embedded in surface regolith (including H2, O2, N2, C, other light atoms, and most tantalisingly, He-3). The ex-astronaut, Harrison Schmitt, with Kulzinski of U of Michigan, have long championed He-3 mining for future fusion reactor power plants, but it is present only at ppb levels. Furthermore, the proposed He-3-D fusion reaction has a ten-times-higher (more demanding) Lawson (Ignition) Criterion than the alternative D-D reaction, so is even further off in terms of availability / TRL. Note however that such mining and extraction of He-3 would automatically make available as byproduct massive quantities of other volatiles for on-moon and off-moon uses.
- Helium-3 production would require some ‘large to humungous scale’ strip mining to access in any sensible quantities.
High grade but rare or quite restricted in locational range:
-
(iii) Metal shards from impact of M-and S-type asteroidal bodies. Apart from the direct utility of the Ni-Fe for on-surface construction use, these are also proposed by Dennis Wingo in his book MoonRush as sources for PGMs for extraction and export to Earth. This is the only realistic ‘PGMs recovery story’ in the entirety of space mining literature. Wingo is pursuing this via his review of old Lunar Orbiter photos from pre-Apollo era, looking for impact shard strewn fields (in a converted ‘MacDonalds’ restaurant at NASA Ames Research Center in San Jose).
-
- (iv) Ice in permanently shaded polar craters. This is the ‘flavour of the decade’ and is the major focus of the recent Collaborative Review based out of Colorado School of Mines led by George Sowers (ex-ULA)and people from various Denver-based organisations including Atomos and ULA. Jim Keravala’s ‘Shackleton Energy’ was also intended to access this resource (before it ran out of money).
- The approaches to design of a mining / extraction system for these different potential resources(ice vs oxygen-from-ilmenite vs shards of Ni-Fe metal vs He-3 etc) must necessarily be very different.
- There is an ongoing debate within the ‘lunar miners’ community’ as to whether lunar polar ice or equatorial mare regolith (for volatiles, or for oxygen and metal) are preferable, and only a slowly dawning recognition (among those who are not professional miners) that very different approaches are required for these two quite different cases. The technologies, TRLs, risk profiles and economics/ scale ability / technical challenges are very different. And the markets are still ill-defined. Do they for example extend to supply of rocket fuel as far down the gravity well as into LEO?
- In contrast to the Moon, NEAs contain orders of magnitude higher grade of all potential valuables (water, other volatiles; Ni-Fe either as reduced metal (in S-type) or as magnetite (in C-cadre); carbonaceous kerogen-like matrix ‘plastics’). OTOH, the Moon (apart from ice in polar craters) is very, very low grade ‘ore’.
- Accessibility as measured by delta-v out and back shows a subset of NEAs which are easier to get to and return from than the Moon; and having low departure d-v and zero gravity means low-thrust non-impulsive thrusting is OK; no need for powerful rocket as in the case of lift-off from the Moon.
- Ice mining in permanently shadowed craters at the lunar poles will (it is thought) provide access to high grade water, but only at extreme low temperatures, and only in very restricted locations in permanently-shadowed craters set by line-of-sight presence of ‘Peaks of Eternal Light’, for placement of solar power panels
- Lunar regolith is amazingly abrasive, due to microscopic glassy fragments, and tightly compacted and very difficult to dig, due to continual shaking by meteorite impacts.
- Telemetry latency time(out-and-back signal time-of-flight) is the major drawback of asteroid operations: thus requires simplicity and local intelligence, and ‘stop-if-in-danger’ control logic.
Moon | Near Earth Asteroids | |
---|---|---|
Moon vs highly-accessible Near Earth Asteroids as Resource Targets | ||
Outbound d - v from LEO | 6 .2 km/s to soft - landing | 18% are < 6km/s to rendezvous ; some ≈ 4 km/s |
Return d-v to Earth-intercept | 2.4 km/s | 0.5 to 2.0 km/s ( best: see Trajectory Browser) |
Gravity well (v - esc) | 2.4 km/s thus needs impulsive thruster | Few cms/sec ( essentially nil) |
Availability H2O in regolith | 50 ppm H2O ( several % in polar craters ? ) | 5 - 20% H2O in average C-cadre asteroids |
Content of metals in regolith | ca. 50 ppm Fe-Ni | 10% in C-cadre; 20 - 50% S - types, 90% M - types |
Complex carbon compounds | no | Yes, in carb. chondrite matrix of C-cadres |
Diggability of regolith | difficult , very resistant | Indications of easy diggability, very low UCS |
Abrasiveness of material | Very aggressive | Likely to be much milder (no glass shards) |
Temperature | Very severe (polar shadow) ca 50 - 100K | 270 K+/- 50 K |
Diurnal (solar) cycle | 14 days, severe energy storage probs | ca. 2 to 10 hours |
Solar power | Full - time only at polar ‘peaks of eternal light’ | OK; but solar concentrator may need to rotate |
Number of targets | Only in permanently shadowed polar craters | Hundreds, depending on selection criteria |
Control constraints | 2 sec latency thus very easy teleoperation; manned setup / intervention possible | Very long latency times: good robotics reqd; manned not feasible in near term |
Engineering challenges | 0.16 g gravity means familiar equipment | Zero- g requires first - principles engineering |
The ‘Sonter-Lewis Hypothesis’ (my terminology) is that ‘90%+ of all NEAs will be either high-grade resource objects for recovery of water, carbonaceous material, and other volatiles (with magnetite as probable co-product), or high-grade resource objects for recovery of native (reduced, metallic) Ni-Fe metal.’
Probabilistic Estimates of Inferred Mineral Resource for water on accessible NEAs have been robustly made -and published, by me- but such estimates cannot yet be made for lunar polar ice deposits, and these asteroid resource estimates support my interpretation of accessible NEAs as ‘mineral resource’ objects, in strict JORC and NI 43-101 terms, even in absence of confirmatory spectroscopic or albedo information.
Accessibility (and therefore regulatory control aspects) are very different between lunar and asteroid resource operations. In case of lunar mining, outside interference is potentially very easy to contemplate, with access to the moon being essentially unlimited, whilst in the case of asteroids, outside interference (eg piracy) is very very difficult, basically because of the self-isolating effect of limited and intermittent launch window opportunities and long synodic periods.